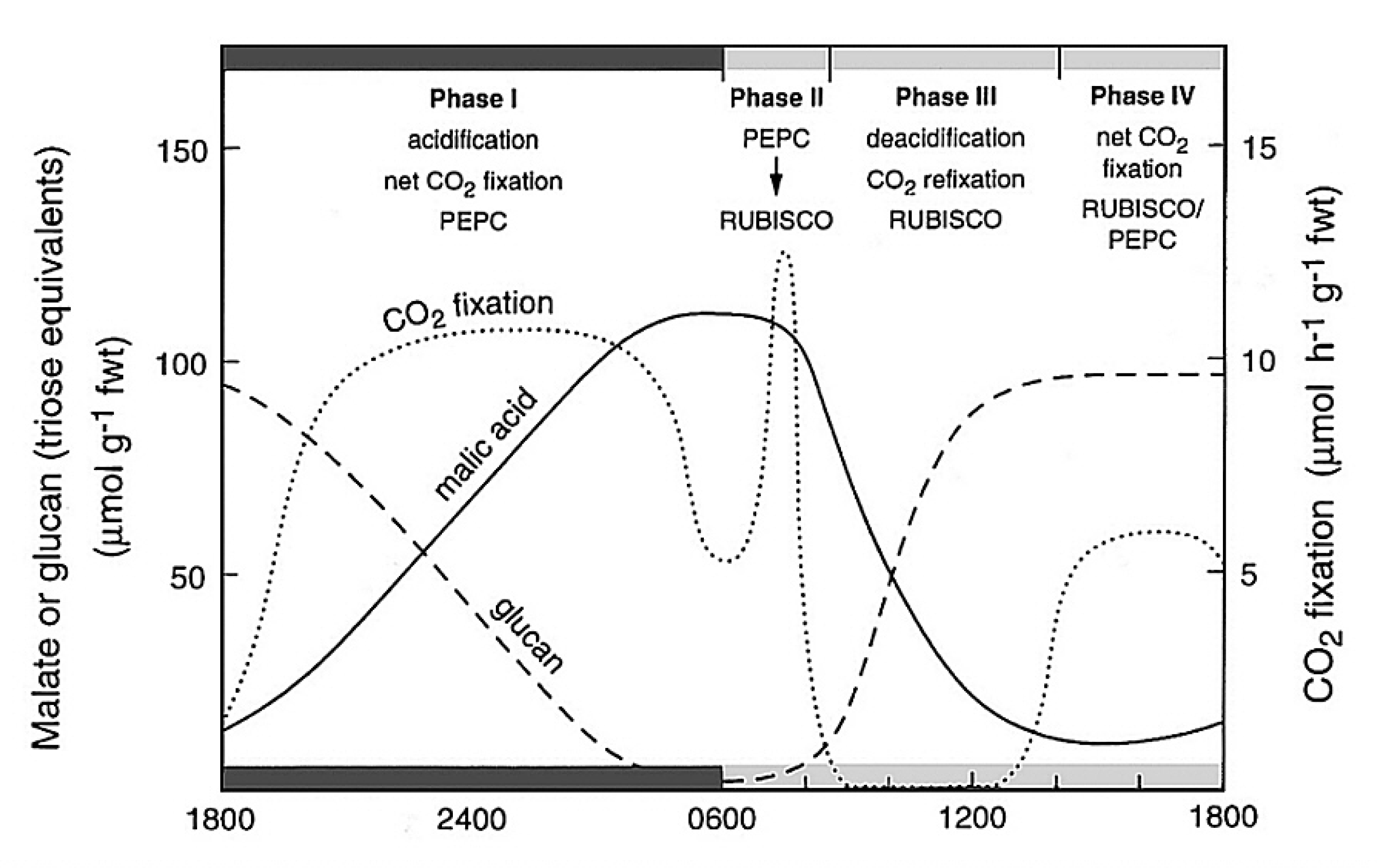
Figure 2.33 Schematic outline of the phases of CAM, showing net CO2 exchange and malic acid and carbohydrate (glucan) metabolism in Kalanchoë daigremontiana leaves. (Diagram courtesy B. Osmond)
Although CAM and C4 photosynthesis share common enzyme machineries, the physiological bases of spatially-separated and time-separated CCMs are very different and involve complex suites of distinctive regulatory processes ranging from allosteric modulation of enzyme activities, through cell and organelle membrane metabolite transport systems, to long-term responses to stress. The resulting metabolism is rarely at steady state. It is thus helpful to reference the principal biochemical interacting components of CAM to the CO2 exchange patterns and the pool sizes of acidity and carbohydrates in the archetypical Kalanchoë daigremontiana as outlined in Figure 2.33.
The four phases of CAM metabolism are:
Within these four phases, the distinctive underlying biochemistry of CAM involves the up-regulation of cytoplasmic PEPC activity during phase I in the dark. Up-regulation is catalysed by PEPC kinase which phosphorylates PEPC making it less sensitive to inhibition by malic acid as it accumulates in the vacuole. Towards night’s end, CO2 fixation by PEPC declines as its carbohydrate substrates are exhausted (Figure 2.33). PEPC kinase is degraded during phase II and PEPC becomes increasingly sensitive to malic acid (declining Ki malate; Figure 2.34). It remains inhibited throughout phases III and IV.
CAM also involves the up-regulation of Rubisco in the light by ATP-dependent Rubisco activase as photosynthetic electron transport (ETR) increases in phase II and is maintained throughout phases III and IV (Figure 2.34).
Partitioning of carbohydrate metabolism occurs in the light to retain chloroplast starch or vacuolar sugars as substrates for the next nocturnal acidification phase (phase I) while diverting sugars for phloem transport and growth. In pineapple, for example, degradation of starch in the chloroplast may provide the substrate for PEPC despite the large diel turnover of soluble sugars. The complexity of this “conflict of interest” (Borland and Dodd 2002) in carbohydrate metabolism varies between CAM plants with different deacidification pathways.
Sophisticated interactions occur between metabolite transporters in membrane systems of the vacuole, mitochondria and chloroplasts. Many of these are unique to CAM but of 48 such transporters required to support known variations of CAM (including Clusia spp. that also accumulate citric acid) up until 2005, only 8 had been demonstrated in at least one species (Holtum et al. 2005).
When studied under constant conditions, many of the above distinctive biochemical processes in CAM exhibit circadian rhythms. The extent to which endogenous oscillators orchestrate the clearly interacting biochemical, physiological and environmental controls seems likely to remain a challenging area of research.