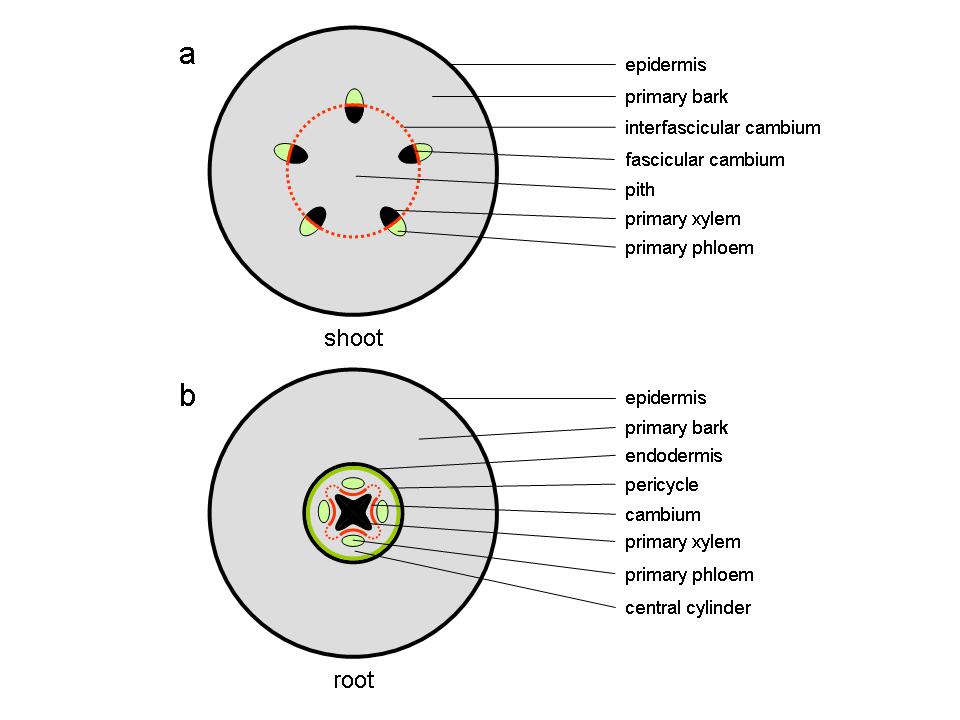
Figure 7.18 Diagram of transverse sections through stem and root at the end of primary growth. (a) dicot stem (b) root.
Gerd Bossinger and Antanas V. Spokevicius, School of Ecosystem and Forest Sciences, University of Melbourne
At the end of the first growing season, the stems of perennial plants consist of a parenchymatous pith in the centre of the stem surrounded by primary vascular tissue within a cortex of parenchyma, collenchyma and sclerenchyma cells, surrounded by an epidermis. The vascular tissue, consisting of xylem towards the inside of the stem and phloem to the outside, appears either as discrete vascular bundles (Figure 7.18a), for example as in Arabidopsis thaliana, or as a continuous hollow cylinder as in eucalypts. In either case, both phloem and xylem cells derive from a layer of meristematic cells forming the fascicular cambium which at the end of primary growth join up with meristematic cells between individual bundles, the inter-fascicular cambium, to form a complete meristematic cylinder, the vascular cambium.
At this stage, the primary root consists of a central column of xylem surrounded by a number of phloem strands formed and separated by procambium tissue. This vascular tissue is contained within a central cylinder that is enclosed by a pericycle and an endodermis, surrounded by primary bark and an epidermis (Figure 7.18b).
How did this develop? And how does cambial growth proceed to form the secondary stem tissues, wood to the inside of the stem and bark to the outside?
The procambium forms early during primary growth (in fact as early as the formation of a heart shaped embryo) and, after germination, it can be found in leaf and primary stem tissue. In the embryos of most dicotyledonous plants, the procambium is established from the differentiation of ground meristematic tissue in the hypocotyl and, as the embryo develops cotyledons, the procambium begins its activity by undergoing acropetal (from the base towards the top) divisions toward the shoot apex. After germination has been initiated and leaf formation begins, the procambium starts to develop further and becomes visible as cell bundles from leaf traces formed below the shoot apex. The procambium then begins to advance acropetally toward the newly derived cells below the shoot apical meristem and, basipetally to connect with the vascular tissue in lower, more mature parts of the stem. As the pro-cambial strands mature a lateral initiating or meristematic layer develops, the fascicular cambium (Figure 7.18a). Cells in this region undergo substantial longitudinal elongation prior to completing their development. While interfascicular cambium differentiation is not fully understood some evidence suggests that it forms between distinct vascular bundles from cells predetermined early in development, which under hormonal control re-differentiate to become meristematic.
Auxin is basipetally transported from leaf primordia to the shoot apex and is believed to be the major factor involved in the process of vasculature patterning and differentiation. The initiation of fascicular and interfascicular cambia is believed to be controlled by different and distinct mechanisms. For example, diffusion of auxin in the form of indole-3-acetic acid is involved in the initiation of meristematic activity and vascular tissue differentiation in the interfascicular region of Arabidopsis thaliana. At the molecular level, a mutation in a Class III homeodomain-leucine zipper (HD-ZIP) gene named INTERFASICULAR FIBERLESS (IFL1) leads to the loss of extra xylary fibre cell formation in the interfascicular region but does not have an effect on vascular bundle formation. This gene is expressed preferentially in the interfascicular region and acts as a transcription factor affecting the polar transport of auxin.
In the root, the cambium develops first from procambial tissue in the grooves between the star-shaped primary xylem and individual primary phloem strands (Figure 7.18b). Soon after, pericycle cells opposite the xylem protrusions divide periclinally (the plane of cell division runs parallel to the surface) and the centripetally (towards the inside) derived daughter cells become part of the cambium, now separating primary xylem from primary phloem.
The cambial cells opposite the primary phloem strands begin to produce secondary xylem transforming the star-shaped cambium into a cambial cylinder gradually producing secondary xylem and phloem around its entire circumference.
The cambial zone includes a tier of meristematic cells between the developing wood (xylem) towards the inside of the stem and bark (phloem) tissue towards the outside (Figure 7.19). Cell divisions in this region either occur in parallel to the stem axis (periclinal) adding cells to a radial file of cells, or perpendicular to the stem axis (anticlinal) adding new cells to the initiating layer and thereby allowing for the establishment of new radial files. The cambium proper is formed by a layer or layers of initiating cells (cambial initials) that undergo mostly periclinal, but also anticlinal division to give rise to radially aligned files of secondary tissue. These initials are enclosed on both radial walls by xylem and phloem mother cells, respectively. Mother cells have a higher differentiation state than initials as they are destined to become either xylem or phloem elements, whereas true cambial initials retain the ability to produce both. Xylem and phloem mother cell divisions make up the majority of periclinal divisions in this zone and cambial initials replenish mother cells when those are lost from the cambial zone. Most anticlinal divisions are contributed by cambial initials resulting in the formation of new radial files and supporting the increase in girth of the growing tree stem.
Meristematic cells in the cambium include fusiform initials and ray cell initials, which are responsible for the formation of specific cell types in both xylem and phloem tissues (Figure 7.19b). Fusiform initials give rise to longitudinally aligned cells including vessels, tracheids and fibres towards the inside of the stem (in gymnosperms only tracheids are formed) and sieve tube elements towards the outside. They are large (compared to ray initials), longitudinally elongated and highly vacuolated. Ray initials on the other hand give rise to transversely aligned cells, primarily ray parenchyma cells, and occur as scattered aggregations within the cambial zone. The ratio of fusiform to ray initials can vary within the cambial zone depending on plant species, age, environmental factors and growth rates. For example, in angiosperms, fusiform initials on average make up 60-90% of the initials within the cambium. While in some species the cambium is comprised entirely of fusiform initials in others they make up as little as 25%.
Fusiform initials in the cambium occur either in a storied arrangement, where cells are laterally aligned, or non-storied, where they are arranged irregularly and often overlap. Divisions in a storied cambium generally occur synchronously resulting in lateral alignment and are typical of some small woody plant species, whereas divisions in a non-storied cambium occur asynchronously and are typical in trees. A storied cambium is believed to represent a more advanced evolutionary arrangement. Fusiform cell derivatives undergo variable amounts of cell expansion during differentiation with radial width often doubling and longitudinal length remaining more or less similar except for some longitudinal extension at the tip (expansion zone in Figure 7.19b). Longitudinal extension, particularly in xylary elements, occurs in the form of intrusive (or sliding growth) which involves the extension of tapered end walls of derivatives into the available space around them. Consequently, the end walls intertwine with end walls of other cells forming strong bonds that increase the mechanical strength of the stem.
The arrangement of rays in angiosperms is highly variable and can be uniseriate (1 cell wide), biseriate (2 cells wide) or multiseriate (3 or more cells wide) while in gymnosperms rays are exclusively uniseriate. Ray initials do not undergo much elongation during differentiation and have been observed to unite frequently, both vertically and laterally to form aggregate rays as well as to split by cell invasion of fusiform initials. The size of ray initials tends to remain constant throughout the life of the plant but their derivatives show a gradual increase in size with age and distance from the pith. Trans-differentiation from a fusiform initial to ray initials can occur in the cambium to maintain a balance between derivatives. This takes place either by segmentation or by progressive shortening of a fusiform initial, whilst trans-differentiation from a ray to a fusiform initial involves elongation via intrusive growth. The mechanisms that drive this process are largely unknown but proximity to other ray initials as well as the effects of plant hormones such as auxin and ethylene have been suggested.
Further differentiation of cambial cells results in the formation of vascular tissues, which are involved mainly in longitudinal transport of liquids and solutes up and down the plant stem. This is facilitated by the creation of long, unbroken tubular structures. Vascular tissue is made up of two distinct elements, xylem and phloem, with xylem being involved in the transport of water, soil derived nutrients and plant hormones from the roots up to the shoots, and phloem in the transport of organic material such as photosynthetic products, proteins, hormones and other regulatory molecules from the leaves to the roots. During secondary growth, these tissues, in particular xylem, are also involved in providing mechanical strength to the stem and as a storage sink for metabolites produced in other parts of the plant. Production of these conduits from the cambium is polarised with xylem derivatives produced centripetally (toward the inside of the meristematic layer) and phloem derivatives centrifugally (toward the outside of the meristematic layer).
Secondary vascular tissue, produced by fusiform initials in the cambium consists of axially aligned tracheary elements (fibres, tracheids, vessels) involved in xylem transport and axially aligned sieve elements (sieve tube cells, companion cells) involved in phloem transport. Ray and axial parenchyma cells are also products of meristematic activity in the cambium and occur in both xylem and phloem tissues. Ray parenchyma cells are aligned radially and axial parenchyma cells are aligned longitudinally. Both parenchyma tissues are involved in transport and storage.
In angiosperms longitudinal transport in the xylem occurs in vessels, which are specialised for liquid transport and arranged end on end to form a continuous, longitudinally aligned tube system. Vessel elements exhibit tapered end walls and thickened lignified cell walls. Prior to maturation and becoming functional, vessel elements undergo programmed cell death (PCD), a process during which cellular content is actively removed, which together with specialised end structures known as perforation plates allows for unimpeded flow between individual vessel elements. Vessels do not form completely straight parallel tubes but rather deviate from their axial path slightly to come in contact with other vessels creating a network of interconnected tubes that allow for movement between vessels through cell wall pits.Vessels are surrounded by longitudinally aligned fibre cells which have a role in providing strength to the plant stem. Compared to vessels, fibres are longer and narrower, have thicker, usually more heavily lignified cell walls, increased tapering of their end walls and they lack a perforation plate. Fibres also undergo PCD as they mature and contain simple pits on their cell walls that allow for minimal transport of liquids between adjacent fibres. Fibres are found most frequently in the xylem and, to a lesser extent, in the phloem where they are arranged in small bundles. In gymnosperms, both transport and strength are provided by a single xylary cell type, tracheids, which, in terms of morphology, are an intermediate form between a fibre and a vessel element. Tracheids are also found in angiosperm stems but to a much lesser degree. Transport of liquids between tracheids is via specialised cell wall openings called bordered pits which through passive opening or closing via acentral, thickened area of pit membrane (torus)can prevent gas or water movement (for example to prevent possible embolism).
Parenchyma cells are rectangular to almost isodiametric in shape and alive at maturity. They are involved in a variety of roles such as transport, signaling, storage and wound responses. Walls of parenchyma cells have a characteristically high number of plasmodesmata that allow for efficient cell to cell communication and transport. Ray parenchyma cells are the only cells within a tree stem that are aligned radially and play an important role in transport and communication between xylem derivatives, cambial initials and phloem derivatives. Similarly, axial parenchyma cells are the only live cells aligned longitudinally in the xylem. They are important for storage and transport in this direction while in the phloem they more often fulfill a storage function.
In the phloem, longitudinal transport occurs via sieve tube elements, which like vessels, are aligned end to end and form continuous tubes. Individual elements are connected by structures called sieve plates. Sieve tube elements undergo some elongation and cell wall thickening during differentiation but differ from vessels and fibres as they do not undergo lignification. Also sieve tube elements are alive at maturity and have adapted a highly modified cytoplasm to allow for free movement of solutes between cells. Here, so called P-proteins internally line all sieve tubes and in the event of wounding can seal sieve plates and allow for wound closure. Cytoplasmic modifications occur via the removal of large organelles including the nucleus, ribosomes, golgi bodies, the tonoplast (vacuoles) and a cytoskeleton as well as links of plasma membranes between longitudinally aligned cells. Sieve tube elements form an association with companion cells, which in angiosperms are derived from a single division of a sieve tube element during differentiation while in gymnosperms they are derived from separate lineages. Companion cells are believed to produce and secrete information molecules via plasmodesmata to associated sieve tube elements.
The most prominent cell division type in the cambial zone, making up approximately 90% of divisions in the cambial zone, is periclinal or additive division, which leads to the formation of highly organised files of radially aligned cells. Unlike in most cell divisions in plants where the cell plate forms across the shortest distance of the cell, cells undergoing periclinal division in the cambium have a cell plate that forms across its long axis. In this process the cell plate begins formation around the mid point of the cell and develops longitudinally until it connects with two lateral cell walls at the end of a cell, resulting in the formation of two nearly identically sized daughter cells. Anticlinal divisions or multiplicative divisions do not occur as frequently as periclinal divisions and are responsible for the formation of new radial files within the cambial zone capable of further periclinal divisions. Anticlinal divisions account for the increasing stem circumference as a result of radial growth as cell wall expansion of initials and their derivatives is not sufficient to compensate for this. This results in a higher frequency of anticlinal divisions during times of accelerated plant growth. The number of anticlinal divisions occurring in fusiform initials is often higher than the number required to compensate for an increase in stem circumference. Superfluous cells are either lost or squeezed out from the cambial zone or undergo trans-differentiation to form ray initials.
Wood formation (xylogenesis) comprises cell division, cell expansion, secondary cell wall deposition, lignification and finally programmed cell death, which occur in highly coordinated and defined ways in response to the environmental conditions imposed on the plant. In mature tree stems, fusiform cambial initials give rise to fibres, tracheids and vessel cells (only tracheids are formed in conifers) while ray cell initials give rise to ray parenchyma cells which, combined, form the ray system. Following division, but prior to the commencement of secondary cell wall deposition, the immediate xylogenic derivatives of fusiform cambial initials (i.e. xylem mother cells) undergo cellular expansion to reach their final form. The extent of this turgor driven expansion is regulated by a range of enzymes that assist in the association and disassociation of primary cell wall components including pectin, randomly aligned cellulose microfibrils (MF) and other non-cellulosic polysaccharides.
The beginning of secondary cell wall formation marks the end of cellular expansion with the production of new cell wall layers leading to cell wall thickening. This stage is characterised by the deposition of large amounts of aligned cellulose microfibrils to the inside of the primary wall in three layers, S1, S2, S3. These differ in their thickness and cellulose MF alignment, and also by the development of cell wall pits. The S2 layer is the most dominant of these layers, accounting for approximately 75-85% of the cellulose content in the secondary cell wall, and as far as wood properties are concerned, has the largest influence. For example ‘Microfibril Angle’ (MFA), one of the most important complex wood quality traits, refers specifically to the cellulose microfibril orientation in this layer of the fibre (or tracheid) cell wall. MFA is known to influence the stiffness and elasticity of individual cells as well as wood and wood products. During this process, the cell wall lignifies and finally undergoes programmed cell death (PCD) during which all cellular content is lost through the action of proteolytic enzymes.
In many tree species, a final developmental process, heartwood formation, occurs later during development. With progressing age, and with the continuous differentiation of some fusiform cambial initials into new vessels, older vessels towards the center of angiosperm tree stems no longer transport water and nutrients and the actively conducting sapwood develops into heartwood. The only cells that are still alive in the sapwood/heartwood transition zone are ray parenchyma cells. Here, they are believed to undergo programmed cell death, translocating polyphenolic substances into surrounding cell walls, leading to the discoloration of heartwood. Where ray cells of some angiosperm trees are directly associated with larger vessels, as a final developmental process, they can produce tylose plugs, decreasing wood permeability and preventing microorganisms from entering the center of the stem. A similar process occurs in sapwood in response to wounding in order to avoid embolism (Figure 7.20).
Figure 7.20 Tylose formation in mature Eucalyptus nitens stems (photos and microscopy by Dr Lawrie Wilson). Left: In the sapwood/heartwood transition zone, tylose plugs are formed by vessel-associated ray parenchyma cells, growing through pit pairs into the vessel lumen, eventually blocking them off from the transpiration stream. Right: Tylose plugs can also form in sapwood vessels in response to wounding. Here, disconnection of an affected vessel from the transpiration stream prevents air from entering the system and cause embolism.
The deposition of lignified secondary cell walls marks the end of cellular expansion and is central to the process of xylogenesis and ultimately determines the functional properties of wood. Secondary cell walls consist of cellulose, lignin and other non-cellulosic polysaccharides which typically represent 90% of the wood dry weight and provide support for the cell. Other minor yet important contributions to cell wall structure and function are made by cell wall proteins and other compounds. Quantitative and qualitative changes in the composition of these elements can lead to changes in cell wall properties and are responsible for the large variation in wood properties observed within and between tree species.
Throughout the year, the cambium undergoes cyclic or induced dormancy and activity periods and wood properties show high levels of variability in response to seasonal, developmental, genetic and abiotic processes. In temperate regions, typically in the northern hemisphere, the vascular cambium undergoes seasonal or periodic dormancy in response to unfavorable and/or detrimental environmental conditions. This dormancy period is usually triggered by a decrease in photoperiod, temperature and water availability during the autumn months preparing the plant for adverse winter conditions and in deciduous plants is closely linked with leaf shed. Prior to the onset of dormancy as conditions begin to decline, cambial activity as well as the number of dividing cells is reduced and cell wall thickness in fusiform initial derivatives in the differentiation zone increases. These seasonal differences in the formation of woody tissues enable the distinction between earlywood (larger cell lumen and thinner cell walls) and latewood (smaller cell lumen, thicker cell walls) leading to the creation of growth rings. Ray initial derivatives on the other hand do not undergo such strong seasonal changes in morphology but their number in the cambial zone decreases during dormancy. Changes in wood properties are also observed between wood produced from early stem growth, juvenile wood, and wood produced later from an older cambium, mature wood. Abiotic stress can lead to reaction wood formation (for example in response to non-vertical growth; referred to as tension wood in angiosperms and compression wood in gymnosperms) displaying altered secondary cell wall characteristics and wood properties to ‘normal’ wood in an attempt by new cambial growth to realign the stem back to a vertical position.
As the plant stem increases in girth during secondary growth, the outermost protective layer of the stem, the epidermis, is gradually shed and replaced by a periderm. Peridermal tissue is produced by the cork cambium (or phellogen) which, like the vascular cambium, comprises a meristematic layer that produces derivatives, both centrifugally and centripetally, and undergoes periclinal and anticlinal division forming radial files of cells. Derivatives from the phellogen differ from those of the cambium both structurally and functionally and the rate of division from this meristem is often much lower than that observed in the vascular cambium. The periderm is comprised of three different tissue types; 1) the phellogen or cork cambium, the meristematic tissue of the periderm, 2) phellum or cork, the outermost protective layer of tissue, and 3) phelloderm, the living parenchyma formed toward the inside of the phellogen. The phellogen is made up of a single layer or tier of cells that are radially flattened, rectangular to polygonal in shape, with thin primary walls, dense cytoplasm and, in some cases, chloroplasts. Phellum cells divide centrifugally from the phellogen and are highly specialised, creating an imperious barrier to gases and liquids on the plant stem. Phellum cells are usually polyhedral in shape, but can be radially compressed and/or elongated in several planes (radial, tangential and longitudinal) and are arranged compactly, lacking intercellular spaces. Phellum cells develop a layer of suberin, an unsaturated fatty acid that impregnates the cell wall during development and gives them their impervious properties. Maturation of these cells ultimately results in cell death. In some genera, e.g. Eucalyptus, phellum cells can also be lignified. Phelloderm cells are living parenchyma cells that divide centripetally from the phellogen and are similar in appearance to the adjacent cortical tissue, being only distinguishable by their radial alignment with cells in the phellogen.
The periderm is continuously replaced during growth and the site and tissue type from which it develops differ between species. Initiation of a periderm occurs either uniformly as a ring around the plant, or in scattered groups which link up later via lateral spreading.
Once the first periderm is ruptured, initiation of the next periderm usually occurs from either cortical tissue or phloem parenchyma whereas later periderms form exclusively from phloem parenchyma. This process is repeated continuously during the life of the plant. The formation of a new periderm displaces a large number of cells, which die and are shed from the stem, thus a tree continues to increase in diameter.