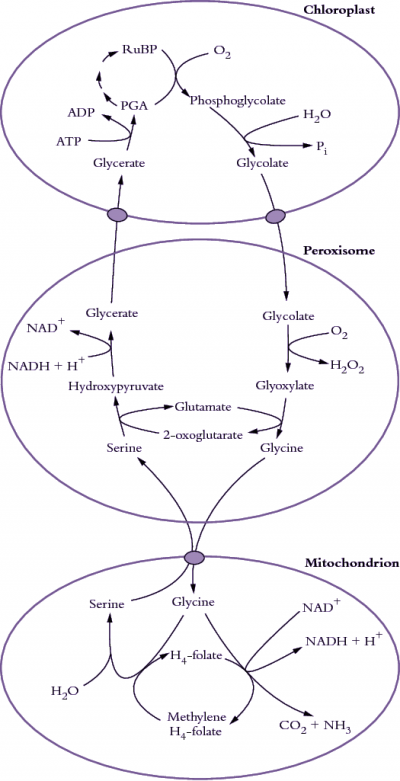
Figure 2.13. The photorespiratory carbon oxidation (PCO) cycle involves movement of metabolites between chloroplasts, peroxisomes and mitochondria. (Original drawing courtesy lan Woodrow)
A scheme for photorespiration involving the photosynthetic carbon reduction (PCR) cycle and the photosynthetic carbon oxidation (PCO) pathways represents the synthesis of almost 70 years of research. It integrates the metabolism of P-glycolate with the PCR cycle. This scheme is shown in Figure 2.13.
Specialised reactions within three classes of organelles in leaf cells are required, namely chloroplasts, peroxisomes (originally called microbodies) and mitochondria. Their close proximity in leaf cells (Figure 2.14 below) plus specific membrane transporters facilitate the exchange of metabolites.
Within choroplasts, oxygenase activity by Rubisco results in formation of phosphoglycolate which then enters a PCO cycle, and is responsible for loss of some of the CO2 just fixed in photosynthesis.
Within peroxisomes, O2 is consumed in converting glycolate to glyoxylate, and aminated to form glycine.
Within mitochondria, CO2 is released during conversion of glycine to serine. Subsequently, serine is recovered by peroxisomes where it is further metabolised, re-entering the PCR cycle of chloroplasts as glycerate. About 75% of carbon skeletons channelled into photorespiration are eventually recovered as carbohydrate.
Transport of glycerate and glycolate across the inner membrane of chloroplasts may involve separate translocators as shown in Figure 2.13, or it may involve a single translocator that exchanges two glycolate molecules for one molecule of glycerate. Transport of metabolites across the peroxisomal membrane most likely occurs through unspecific channel proteins, similar to those in the outer membranes of mitochondria and chloroplasts. These outer membranes are not included in this diagram. Mitochondria take up two molecules of glycine and release one molecule of serine. A specific translocator most probably mediates the exchange of these amino acids.
Not only does the photosynthetic oxidation pathway consume O2 and release CO2 but ammonia is also produced by mitochondria during synthesis of serine from glycine (Figure 2.13). This ammonia would be extremely toxic if it were not metabolised by either cytosolic or chloroplastic glutamine synthetase. A very effective herbicide that blocks glutamine synthetase has been developed (phosphinothricin, also known as glufosinate or Basta), and when it is applied to (or expressed in) actively growing plants they are killed by their photorespiratory ammonia release.
Figure 2.14. A transmission electron micrograph showing close juxtaposition of chloroplast (C), mitochondrion (M) and peroxisome (P) in a mesophyll cell of an immature leaf of bean (Phaseolus vulgaris). This group of organelles is held within a granular cytoplasmic matrix adjacent to a cell wall (CW) and includes a partial view of a small vacuole (V). Scale bar = 1 µm (Electron micrograph courtesy Stuart Craig and Celia Miller)
In summary, participation of photorespiration in leaf gas exchange, and thus dry matter accumulation by plants, reflects kinetic properties of Rubisco, and in particular a relatively high affinity for CO2 (Km = 12 µM) compared with a much lower affinity for O2 (Km = 250 µM). That contrast in affinity is, however, somewhat offset by the relative abundance of the two gases at catalytic sites of the enzyme where the ratio of O2:CO2 partial pressures approaches 1000:1! Thus, CO2 assimilation always prevails over CO2 loss in photorespiration.