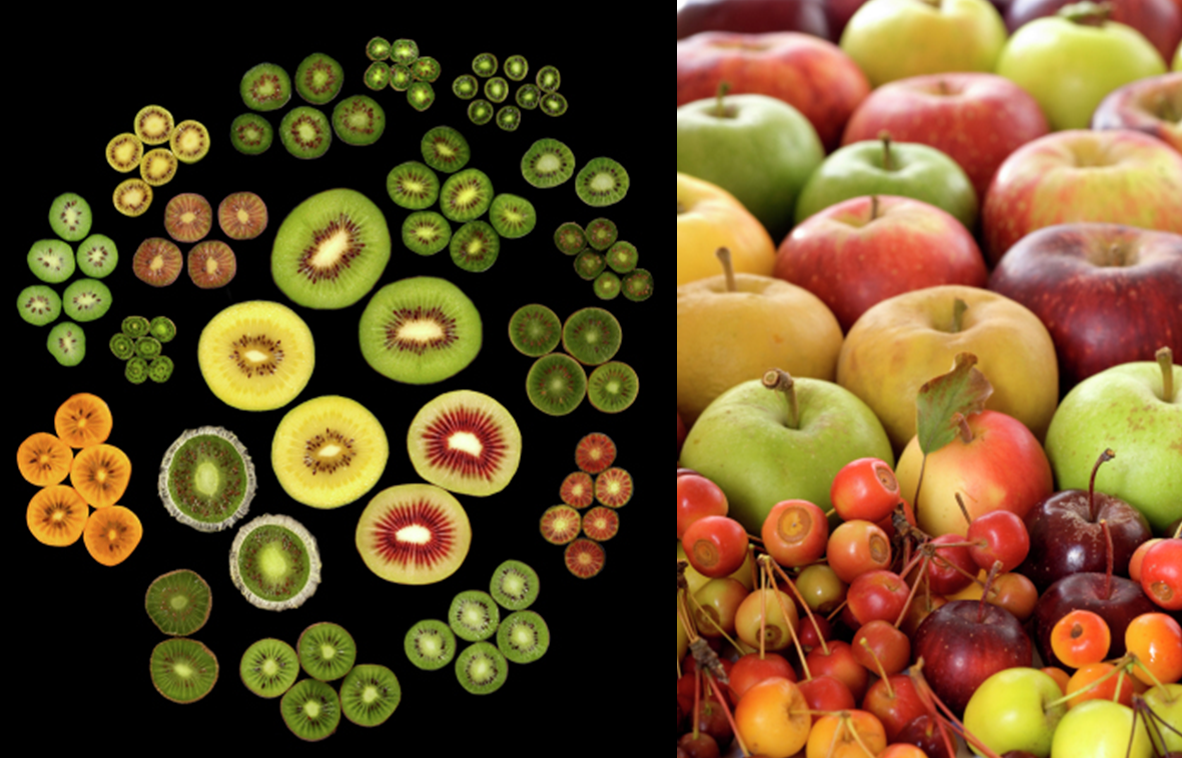
Figure 11.18. Colour diversity in ripe kiwifruit and apple is determined by the presence or absence of chlorophyll, carotenoid and anthocyanin compounds in different fruit tissues. (Photographs courtesy Plant & Food Research)
During ripening many fruit change colour. Their bright colour, which evolved to attract dispersal agents such as birds, browsing animals and primates, has now become a particularly important visible indicator of maturity and ripeness. Bananas, berryfruit and stonefruit provide good examples where colour is a prime indicator of ripeness. Novel colours are also used to market new varieties to consumers, e.g. in kiwifruit, green-fleshed Actinidia deliciosa ‘Hayward’ vs. yellow-fleshed A. chinensis ‘Hort16A’ (Figure 11.18).
By analogy with senescence in most green tissues such as leaves, colour change in fruit typically involves chlorophyll loss and an increase in production of yellow, orange, red or purple pigments. In green-fleshed ‘Hayward’ kiwifruit chlorophyll is retained in the flesh of ripe fruit, whilst in yellow-fleshed ‘Hort16A’ chlorophyll is degraded during ripening by catabolic enzymes in the chlorophyll degradation pathway. This suggests that in ‘Hayward’ fruit chloroplasts are not converted to chromoplasts as is typical for ripening fruit.
The gold, orange and red colours of many fruit such as tomato and citrus are formed by enzymes in the carotenoid biosynthetic pathway (Tanaka et al. 2008). Carotenoids are divided into two classes: the hydrocarbon carotenes, e.g. lycopene (red) and b-carotene (orange); or the oxygen-containing xanthophylls, e.g. lutein (yellow). Besides providing highly attractive pigmentation, carotenoids protect the plant’s photosynthetic apparatus from excessive light energy and are essential requirements for human and animal nutrition.
Other red and purple pigments of the type seen in grapes and boysenberries are anthocyanins, which are products of the phenylpropanoid pathway. Anthocyanin pigments are water-soluble, synthesised in the cytosol and localised in vacuoles. Their basic ring structure can be modified by hydroxylation, methylation or glycosylation and their specific colour is modified by pH, metal ions and co-pigments to produce the subtlety of colours seen in nature. Like carotenoids, anthocyanins have many human health benefits and are widely used as natural food colourants (Tanaka et al. 2008).
The accumulation of anthocyanins is regulated by transcription factors of two classes (R2R3 MYB and basic helix loop helix), regulatory proteins that co-ordinate gene expression of the whole phenylpropanoid pathway. In fruit, this regulation system has been well characterised in grape and apple. In white berry grapes, VvMYBA2 is inactivated by mutations in the coding region and VvMYBA1 has a retrotransposon in the promoter and is not transcribed (Kobayashi et al. 2004; Walker et al. 2007). In apple fruit, a mini-satellite repeat structure in the promoter region of the MYB10 gene up-regulated the expression of this regulatory gene, which increased the level of anthocyanin throughout the plant producing a fruit with striking red colour throughout the flesh (Espley et al. 2009).
Flavour is the most important factor determining if consumers will repurchase a particular fruit. Therefore, all varieties are produced and stored to maintain the very best flavour and aroma properties. Two main factors determine a fruit’s characteristic flavour – the correct sugar/acid balance and the production of aroma volatile compounds. These volatile compounds can include a mixture of volatile acids, aldehydes, alcohols, esters, terpenoids and aromatics.
Human taste sensations and experiences play an important part in characterising volatile compounds in fruit and wine, so a vocabulary has been developed to describe their sensory nature. The terms used relate a particular flavour sensation to that of a widely available standard, and have led to terms like ‘woody, ‘grassy’, ‘floral’, ‘spicy’ and ‘citrus’ (Figure 11.19).
With advanced GC-MS techniques many ripe fruit can be shown to contain >100 volatile compounds that contribute to their flavour and aroma. However, the absolute concentration of a volatile compound itself does not determine how important it is to perceived flavour and aroma. Sometimes compounds found at very low levels, e.g. parts per billion, are required to give a fruit its characteristic aroma. Compounds are given odour activity values (compound concentration divided by the minimum concentration that can be detected by the human nose) to show their importance to aroma.
Sometimes, one or two key volatile compounds can be regarded as characteristic for fruit of a given species or cultivar and are used in synthetic mixtures to represent that commodity. Specific volatiles are especially important in wine grapes where an individual volatile can become the dominant characteristic used in marketing a specific wine type. Examples include the ‘grassy’ character of methoxypyrazine in Sauvignon Blanc, the ‘richness’ of b-damascenone in red wine, or the ‘foxy’ character of methyl anthranilate produced by Vitis labruscana. Other examples of important volatiles in fruit include: raspberry – raspberry ketone; ‘Hort16A’ kiwifruit – ethyl butanoate and 1,8-cineole; ‘Hayward’ kiwifruit – (E)-2-hexenal and hexanal; apple — 2-methyl butyl acetate and a-farnesene, and strawberry — furaneol.
Our understanding of how flavour compounds are synthesised has rapidly advanced in the last 20 years. Aldehydes, acids and esters are derived from fatty acids and branched chain amino acids. The first committed step in straight-chain ester production is performed by lipoxygenases that produce 13-hydroperoxide linoleic acid from linoleic acid. These compounds are converted by cytochrome p450 lyases to aldehydes such as hex-3-enal and hex-2-enal. Alcohol dehydrogenases (ADHs) can then transform aldehydes to the corresponding alcohols, which contribute ‘green’ aromas. Alcohol acceptor substrates are then esterified with coenzyme acid donors by alcohol acyl transferases (AATs) to form esters, which generally contribute ‘fruity’ and ‘sweet’ characteristics. In apple, the enzyme MpAAT1 can produce a range of esters in ripe fruit and is up-regulated by ethylene during ripening (Souleyre et al. 2005). Branched chain esters are produced from isoleucine by branch chain aminotransferases and then pyruvate decarboxylase to produce aldehydes. These aldehydes are then available for ADHs and AATs to form branched chain alcohols and esters, respectively.
Sesquiterpenes and monoterpenes also contribute to fruit flavour and aroma profiles, often by adding ‘floral’ or ‘spicy’ top notes. In apple, the most important ripe-fruit terpene is a-farnesene produced by the sesquiterpene synthase AFS1. ‘Hort16A’ kiwifruit produce 1,8-cineole and A. arguta (baby kiwifruit) produce a-terpinolene, which add spicy/minty notes to these fruit. Terpenoid compounds are produced by terpene synthase enzymes, using farnesyl diphosphate (FPP) and geranyl diphosphate (GPP) as substrates. FPP and GPP are produced in plants by the action of short chain prenyltransferases in two compartmentally separated pathways (Lichtenthaler et al. 1997). In the plastid, the MEP (2-C-methyl-D-erythritol 4-phosphate) pathway leads to the production of GPP, while in the cytoplasm the mevalonate pathway provides precursors for FPP formation. The primary terpenoid skeletons can subsequently be modified further, e.g. by oxidation, hydroxylation, glycosylation or methylation by a range of other enzymes to increase terpenoid diversity.