Interception of light by a crop canopy is strongly related to total or canopy leaf area. A crop will thus intercept more light and hence grow faster if it develops the canopy leaf area rapidly. This principle applies to both annual crops, which are usually planted at the beginning of a growing season and to perennial crops, which resume growth after a dormant season. Leaf area development of sugar cane, for example, is generally slower in the year of planting compared with a subsequent ratooned crop, where canopy regrowth is enhanced by stored photoassimilates. By analogy with early canopy expansion, retention of green leaves late in a growing season also extends light interception and enhances storage of photoassimilates. This is also true for perennial crops. For some deciduous horticultural crops, leaf area expansion is also rapid because of preformed primordia which emerge rapidly and comprise the largest leaves (Greer 1996).
The leaf area index (LAI) is the ratio of total projected leaf area (one side only) per unit ground area, and is widely used to characterise the canopy light climate. A canopy where LAI equals 1 has a leaf area equal to the soil surface area on which it grows. This does not mean all light is intercepted, because some leaves overlap, leaving gaps. Moreover, not all leaves are positioned at right angles to the incident radiation. A crop under favourable growing conditions increases LAI rapidly during early development to a maximum of 3 to 7.
An example of LAI development of three tropical cereal crops grown under well-watered conditions in South East Queensland is given in Figure 12.19. Sorghum showed a more rapid increase in LAI than did maize, largely because of a higher sowing density (33 v. 5.6 plants m–2). A late maturing rice crop showed slowest leaf area development during early stages of growth, but the maximum LAI was none the less higher for rice than for maize. As a general rule, maximum LAI is achieved just prior to flowering in cereal crops. By that stage, growing points are differentiating floral rather than leaf primordia, and initiation of new leaves has ceased.
Some cereal crops lose leaves and the LAI declines during grain filling as crops mature. Differences in LAI development among the three crops (Figure 12.20a) are evident in light interception by the respective canopies (Figure 12.19b); interception prior to 60 d was highest in sorghum and lowest in rice. However, in all three crops, canopy light interception increased rapidly during early stages of growth. Incident radiation was almost completely intercepted once a high LAI had been achieved.
Despite wide variation in crop phenology, sunlight interception and LAI maintain a tight curvilinear relationship (Figure 12.19c). Thus, interception increases sharply with increases in LAI to about 90% once LAI exceeds 4, and approaches an asymptote at higher LAI, see also Figure 12.20 from Khurana and McLaren (1982). In this research on potato, numerous treatments were imposed, involving different storage of seeds at low temperatures including apically and multisprouted seed treated at 4 and 12 °C and then at 8 °C before planting. In Treatment1, the trial included unsprouted seed stored at 4 °C, in Treatment 2, the trial included seed stored in the dark and in Treatment 3, two additional sprouting treatments were mixed alternately along the row. Such a relationship between LAI and light interception applies to many crops, and emphasises (1) the importance of a rapid increase in LAI during early stages of growth, and (2) a requirement for only moderate LAI to achieve effective light interception. Indeed, excessive leaf area development can be counter-productive, because reproductive development, and hence economic yield, may be reduced due to self-shading and resource allocation to leaf production (such as for fruit trees and grapevines, Section 12.4).
12.2-Ch-Fig-12.20.png
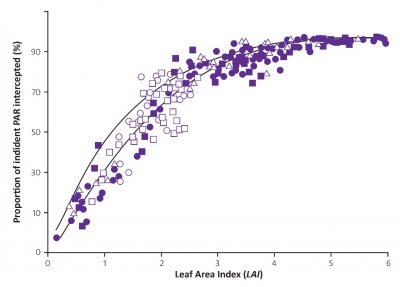
Figure 12.20 The proportion of incident radiation intercepted by potato canopies as a function of leaf area index. The different symbols indicate agronomic treatments over two growing seasons. In 1979: □ cv. Record, ○ cv. Pentland Crown. In 1980: cv. Pentland Crown with three treatments (see text): ●Treatment 1, ▲Treatment 2, ■ Treatment 3. (Based on Khurana and McLaren 1982)
The time-course of light interception during crop growth can be manipulated to some extent by farmers. For example, seeding rate is an important management option which affects interception and subsequent crop growth and yield. A high seeding rate would produce a high plant population density and a high LAI at crop establishment. This hastens canopy interception and hence biomass production would be promoted. Any advantage of a high plant density may, however, disappear with time during crop growth, because radiation interception of a medium plant density may eventually catch up with that of the high density (Figure 12.21).
In this case, density 3 (35 plants m–2) was sufficient for radiation interception and plant dry matter production. If plant density is very low, shown as density 1 (1.4 plants m–2) or density 2 (7 plants m–2) in Figure 12.21, LAI never exceeded 2 and final biomass at harvest was much smaller than values returned from higher densities. Solar radiation was not fully intercepted and hence wasted at low planting density, and potential yield (dry mass produced per unit area) was never realised.
As solar radiation penetrates a crop canopy, PAR is intercepted by leaves and photon irradiance commonly declines exponentially with cumulative leaf area (i.e. depth in Figure 12.23), according to the exponential relationship:
\[ I = I_0 e^{-kL} \tag{12.1} \]
where \(I\) is horizontal photon irradiance within a canopy, \(I_0\) is horizontal photon irradiance above that canopy, \(L\) is LAI from the top of the canopy to the point where \(I\) is determined, and \(k\) is an extinction coefficient (a more explicit formulation for PAR attenuation through a forest canopy is given in the next section).
Large \(k\) values imply that photon irradiance decreases rapidly with depth, whereas a canopy with a small \(k\) would allow solar radiation to penetrate deeply, for a similar leaf area profile. Variation in \(k\) value is commonly associated with leaf angle. Canopies with more horizontal leaves, such as sun-flower or cotton, have large \(k\) values, often 0.7–1.0, whereas those with more erect leaves, such as barley and sugar cane, have small values, often 0.3–0.6.Irrespective of the canopy extinction, there is a strong relationship between the light intercepted by the canopy over the growing season and the total dry matter produced for a number of crops (Fig. 12. 22). Thus, it is imperative on growing crops to manage the LAI to achieve maximum light interception from the early part of the season to maximise production of dry matter.