Min Chen
School of Biological Sciences, University of Sydney, Australia
Fig_1.1.png
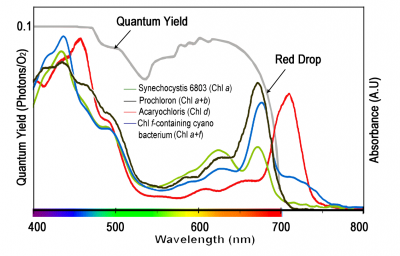
Figure 1. Absorption spectra of photosynthetic organisms containing different chlorophylls and the quantum yield of photosynthesis using chlorophylls a and b (grey line). Green line, in vivo absorption spectrum of Synechocystis PCC 6803 in BG11 medium; Black line, isolated Prochloron cell suspension in seawater; Red line, in vivo absorption spectrum of Acaryochloris marine MBIC11017 in seawater medium; and Blue line, in vivo absorption spectrum of Halomicronema hongdechloris in seawater medium.
Solar radiation is a black body at a temperature of ~5800oK, covering all spectral regions. However, all known eukaryotic photosynthetic organisms (including plants and algae) are only able to use the same region of the solar spectrum that our eyes are sensitive to, covering the wavelength of 400 – 700 nm region, which is approximately 43% of the total solar radiation. This region is called photosynthetic active radiation (PAR) with estimated photon flux of 1.05 x 1021 photons m-2 s-1. Longer wavelengths up to 1000 nm can drive anoxygenic photosynthesis but not oxygenic (oxygen evolving) photosynthesis. The reason for the high threshold energy for oxygenic photosynthesis is the higher energy requirement for catalysing water oxidation and oxygen evolution in photosynthesis. The PAR input limit depends on the absorption of the photopigments. Chlorophylls a and b, the main chlorophylls in eukaryotic photosynthetic organisms, show their maximal absorption bands in the blue region of 430 - 455 nm and the red region of 645 -670 nm, thus leaving a “green window” and photons outside of “visible region” (Figure 1). The photons collected by chlorophylls a and b provide a strong enough redox potential for the oxidisation of water, while at the same time they also provide an negative enough excited state redox potentials for the reduction of the primary electron acceptor.
There are five different chlorophylls that have been identified, chlorophylls a, b, c, d and f. Here, we focus on chlorophylls containing five rings (macrocycle) and an esterified 17-propionic acid side chain, the chlorin type chlorophylls, including chlorophylls a, b, d and f. The chemical difference among the different chlorophylls is either formyl substitution at the side chain of the macrocycle (chlorophyll b, d, and f) or the degree of unsaturation of the macrocycle (8-vinyl chlorophyll a and 8-vinly chlorophyll b). Those chemical structural differences are also the spectral determinants and responsible for the different absorption spectral features (Figure 2).
Fig_1.2.png
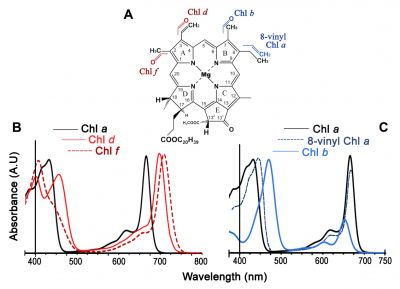
Figure 2. Chemical structure of chlorophylls and their absorption spectra in 100% methanol. (A) Chemical structure of chlorophyll a and the structural differences of other chlorophylls from chlorophyll a. The carbon atoms are numbered using IUPAC system. (B) Absorption spectra of red-shifted chlorophylls, chlorophylls d and f, compared with chlorophyll a. (C) Absorption spectra of chlorophyll b and 8-vinyl chlorophyll a compared with chlorophyll a. (modified from reference 3)
Chlorophyll b is distinguished from chlorophyll a by a formyl instead of a methyl group on ring B at C7 position, which results in a blue-shift of the longest red absorbance band (Qy) from 665 nm to 652 nm. Chlorophyll d and chlorophyll f are distinguished from chlorophyll a by replacement of a peripheral substituent on ring A by a formyl group at C3 position and C2 position, respectively (Figure 2). The consequences of those formyl group substitutions at ring A result into a red-shifted Qy absorption wavelength, from 665 nm to 696 nm (chlorophyll d) and even further to 706 nm for chlorophyll f. Both chlorophylls d and f are named as red-shifted chlorophylls. Those red-shifted chlorophylls allow the organisms to use the light beyond 700 nm efficiently compared with the organisms containing chlorophylls a and b only.
Plants using chlorophyll a and b demonstrate the decreased quantum yield of photosynthesis using wavelength >700 nm, which is known as the “red drop” (Figure 1). The reason for the red-drop at ~700 nm is that the chlorophylls that absorb longer wavelength light beyond 700 nm will not do photosynthesis as efficiently as the chlorophylls that absorb shorter wavelength light. The 700 nm photons were considered as the red-edge of oxygenic photosynthesis. However, the newly discovered chlorophyll d-containing Acaryochloris marina and chlorophyll f-containing Halomicronema hongdechloris have forced a re-evaluation of what is the minimum threshold energy for oxygenic photosynthesis. Both red-shifted chlorophyll-containing cyanobacteria are found in the environment where visible lights are depleted by above layers of oxygenic photosynthetic organisms. The red-shifted chlorophylls allow them to absorb the longer wavelength light beyond 700 nm and do oxygenic photosynthesis as efficiently as above layers of chlorophyll a-containing organisms. Accordingly, the minimum threshold energy for oxygenic photosynthesis has been at extended to at least 750 nm in those red-shifted chlorophyll-containing organisms. The PAR increment in the region of the solar spectrum of 700 – 750 nm increases the number of available light energy by 19%. The potential additional photon flux in the infrared region (700-750 nm) could improve the light-harvesting efficiency by extending the PAR coverage to 400-750 nm if those red-shifted chlorophylls could be introduced into plants and algae.
In addition for the potential enhancement for efficient light collection and transfer to the reaction centres under weak irradiation, the second functional demands for the light-harvesting process is their protecting function at exposure to strong light, which will be covered in a following case study.
Further Reading
Chen M, Blankenship RB (2011) Expanding the solar spectrum used by photosynthesis. Trends Plant Sci 16: 427-431
Chen M, Schliep M, Willows R, Cai Z-L, Neilan BA, Scheer H (2010) A red-shifted chlorophyll. Science 329: 1318-1319
Chen M, Scheer H (2013) Extending the limit of natural photosynthesis and implications of technical light harvesting, J Porphyrins Phthalocyanines 17: 1-15