(a) Delineating the transport path
Phloem loading is used variously to describe transport events outside, and inside, phloem tissues of leaves. The broader general application is adopted here — that is, phloem loading describes photoassimilate transport from the cytoplasm of photosynthetic mesophyll cells to se–cc complexes of leaf phloem.
Phloem loading commences in mesophyll cells and ends in the leaf vascular system. The se–cc complexes occur in a wide array of vascular bundle sizes. In dicotyledonous leaves, veins undergo repeated branching, forming the extensive minor vein network described in Section 5.2. For example, sugar beet leaves contain 70cm of minor veins cm–2 of leaf blade, while the major veins contribute only 5.5cm cm–2 of leaf blade (Geiger 1975). These observations and physiological studies (van Bel 1993) show that the principal site of phloem loading is in the minor vein network of dicotyledonous leaves. In contrast, the major veins transport loaded photoassimilates out of leaves.
Fig 5.22-ann.jpg
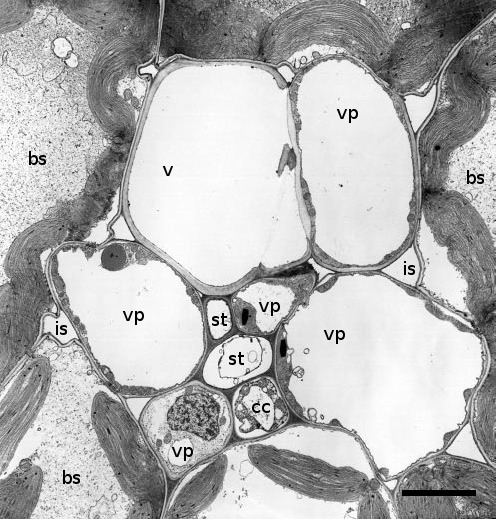
Figure 5.13. Transmission electron micrograph through a minor vein of a source leaf of maize (Zea mays L.) This vascular bundle consists of two sieve elements (st), one xylem vessel (v) and five vascular parenchuma cells (vp). These sieve elements are of two types, one thin walled and accompanied by a companion cell, the other thick walled and adjacent to the xylem vessel. Other symbols are: bs, bundle sheath; cc, companion cell; is, intracellular space; st, sieve tube. Scale bar - 4.2 µm (Based on Evert et al. 1978; reproduced with permission of Planta)
Minor veins usually comprise a single xylem element, vascular parenchyma cells and one to two sieve elements surrounded by one to four companion cells (Figure 5.13). The se–cc complex in minor veins bears similarities to that of stems (Figure 5.6). Companion cells have dense cytoplasm containing many mitochondria and are often considerably larger than the sieve elements they accompany. Companion cells are symplasmically connected to the sieve elements by branched plasmodesmata.
Cross-sectional areas of veins in monocotyledonous leaves reveal large and small parallel veins. Photoassimilates are loaded into the small veins and conducted through large veins. Fine transverse veins carry photoassimilates loaded into small veins across to large veins for export.
(b) Cellular pathways — symplasmic versus apoplasmic
Fig_p_5.23.png
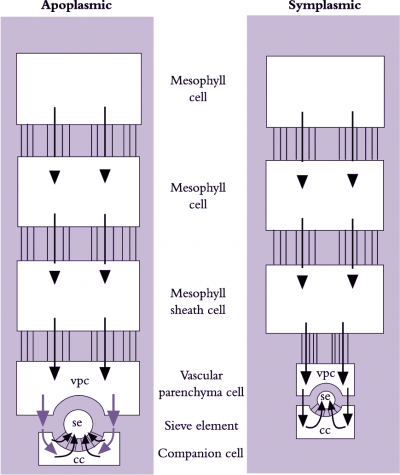
Figure 5.14. Scheme describing symplasmic and apoplasmic pathways of phloem loading. Lines without arrows joining boxes represent symplasmic continuity (i.e. plasmodesmata). Black arrows indicate symplasmic transport (i.e. through plasmdesmata); green arrows indicate apoplasmic transport requiring solutes to cross membranes. vpc, vascular parenchyma cell; se, sieve element; cc, companion cell (Based on van Bel 1993)
Photoassimilates could move intercellularly through interconnecting plasmodesmata from chloroplasts in mesophyll cells to the lumena of sieve elements (symplasmic phloem loading) or across plasma membranes, travelling part of the route through the cell wall continuum (apoplasmic phloem loading). These fundamentally different pathways are shown schematically in Figure 5.14. Debate persists over which cellular pathway of phloem loading prevails because experiments on transport from mesophyll cells to sieve elements are difficult.
Extraordinarily, the cellular pathway of phloem loading reflects evolutionary relationships. Species from ancient plant groups display symplasmic loading, while species of more modern plant groups appears to exhibit apoplasmic phloem loading (van Bel 1993). Evidence for respective routes of loading follows.
A symplasmic pathway depends upon development of extensive plasmodesmal interconnections between adjoining cells, forming a cytoplasmic continuum from mesophyll to se–cc complexes (Figure 5.14). Such symplastic continuity is found in leaves of plant families containing trees and shrubs as well as cucurbits such as squash (van Bel 1993). An abundance of plasmodesmal interconnections demonstrates potential for symplasmic transport but does not establish whether such transport actually occurs. Membrane-impermeant fluorescent dyes microinjected into mesophyll cells are transported to se–cc complexes, demonstrating that plasmodesmata can provide a route for photoassimilate transport. Furthermore, when leaves were fed14CO2 and treated with inhibitors that block sugar transport across plasma membranes, transport of 14C-labelled photo-assimilates continued unaffected along the enforced symplasmic unloading route (Figure 5.15; van Bel 1993). In this case, sugar levels are higher in the mesophyll than in the phloem and ions and molecules diffuse through plasmodesmata at each interface, without a concentrating step (Turgeon 2010). Therefore, this is a passive symplasmic phloem loading.
Symplasmic phloem loading may also be an active process occurring in some herbaceous eudicots. This model of phloem loading, called polymer trap mechanism, depends on sucrose being biochemically converted to raffinose oligosaccharides (RFOs) in specialized CCs (intermediary cells - ICs) (Turgeon 2010). The biochemical synthesis of RFOs from sucrose requires metabolic energy. The synthesized RFOs exceed size exclusion limits of plasmodesmata linking mesophyll cells with ICs and therefore are trapped and accumulate to high concentrations in SE/IC complexes of minor veins for long distance transport (Turgeon 2010).
Plant species that load phloem from the leaf apoplasm are characterised by a low abundance of plasmodesmata between se–cc complexes and abutting vascular cells. However, as for symplasmic loaders, mesophyll cells of these species are interconnected by abundant plasmodesmata (Figure 5.23). Herbaceous and many crop species belong to this group of phloem loaders, including grasses (van Bel 1993). Conventional physiological observations are consistent with phloem loading in leaves of these species including a membrane transport event located somewhere between mesophyll cells and the se–cc complexes of minor veins (Figure 5.15).
Fig_p_5.24.png
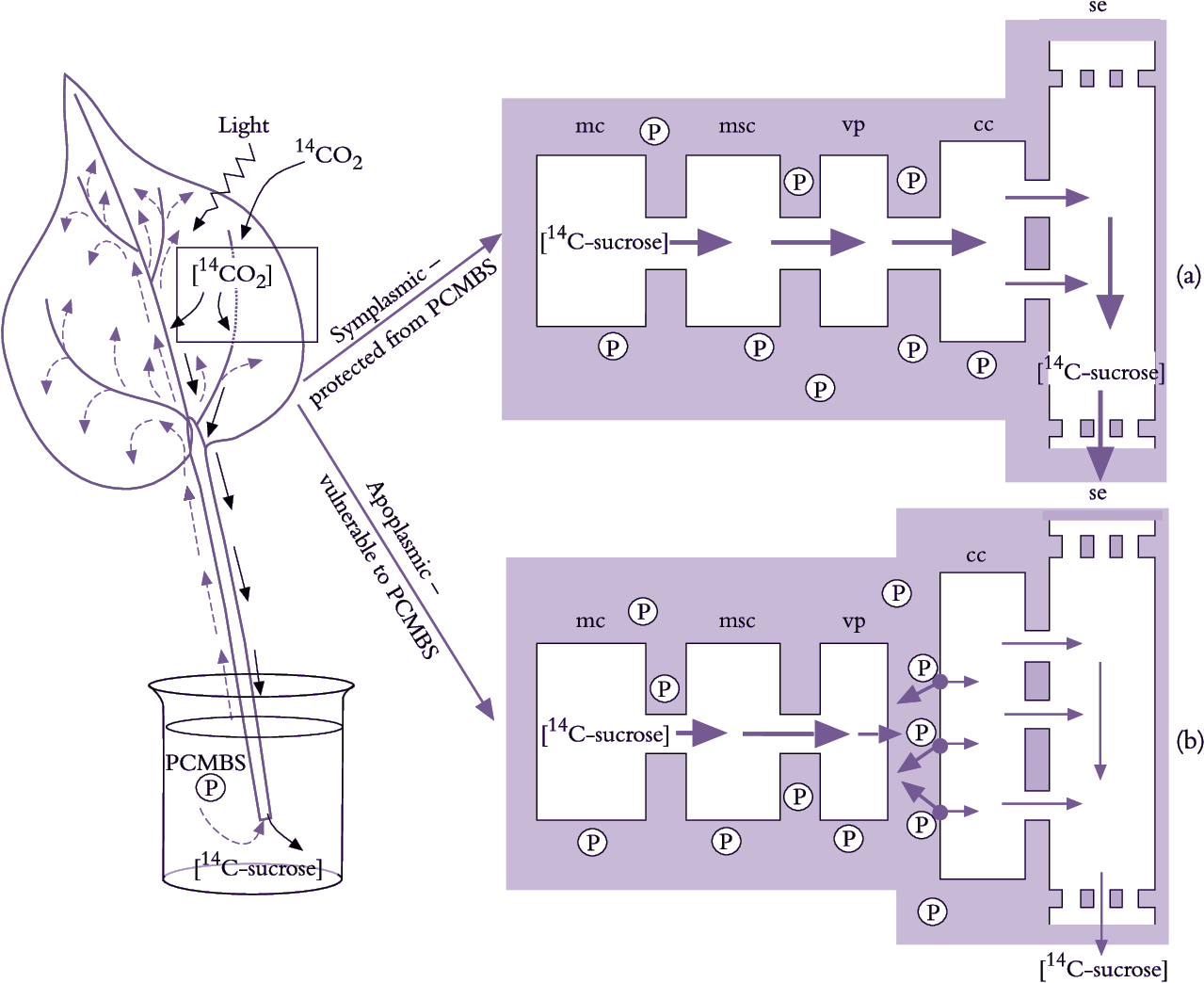
Figure 5.15. Testing whether photoassimilates move from mesophyll cells to se-cc complexes through (a) an entirely symplasmic route or (b) a route with an apoplasmic step. The approach is to use PCMBS as an inhibitor of membrane transport. PCMBS does not cross membranes but binds to the apoplasmic face of plasma membranes. Therefore, it blocks apoplasmic transport while symplasmic phloem loading is unaffected. PCMBS was introduced into the leaf apoplasm through the transpiration stream of excised leaves. Leaves were then exposed in a closed illuminated chamber to 14CO2. The 14C photoassimilate exported from labelled leaf blades was used to monitor phloem loading. PCMBS only reduced photoassimilate export (i.e. phloem loading) from those leaves with few plasmodesmata interconnecting se-cc complexes with surrounding cells. Thus, photoassimilate flow included a membrane transport step from the leaf apoplasm in certain plant species while others loaded via a symplasmic route. cc, companion cell; mc, mesophyll cell; msc, mesophyll sheath cell; PCMBS (para-chloromercuriben-zenesulphonic acid, also abbreviated to P); se, sieve element; vp, vascular parenchyma (Based on van Bel 1993)
Molecular biology has brought new insights to phloem loading. For instance, existence of an apoplasmic step demonstrated with PCMBS (Figure 5.15) has been elegantly confirmed using molecular biology to control activity of the sucrose/proton symporter responsible for sucrose uptake from phloem apoplasm into se–cc complexes. Specifically, potato plants were transformed with an antisense copy of the gene encoding the sucrose/proton symporter, producing a phenotype with low levels of the symporter in plasma membranes of se–cc complexes (Frommer et al. 1996). Excised leaves of transformed plants exported significantly less photoassimilates than wild-type plants, corroborating the inhibitory effect of PCMBS on apoplasmic phloem loading (Figure 5.15). This provides compelling evidence that passage of photoassimilates from mesophyll cells to se–cc complexes in potato leaves includes an apoplasmic step.
Vascular parenchyma cells are the most probable site for photoassimilate exchange to phloem apoplasm (van Bel 1993), ensuring direct delivery for loading into se–cc complexes. Furthermore, plasma membranes of se–cc complexes in minor veins have increased surface areas to support photoassimilate transfer from phloem apoplasm. Notably, the surface area of se–cc complexes in sugar beet leaves is surprisingly large—0.88 cm–2of leaf blade surface. By implication, these large membrane surfaces are involved in phloem loading. Further support comes from cytochemical studies, demonstrating a great abundance of proteins associated with energy-coupled sucrose transport (Section 5.3.3(b)).
Leaf anatomies in some plant species suggest a potential for simultaneous phloem loading through apoplasmic and symplasmic pathways (van Bel 1993). Whether these pathways connect the same sieve element, different sieve elements in the same minor vein order or sieve elements in different vein orders is still unknown.