Leaves are covered with a barrier or ‘cuticle’ on the outer walls of epidermal cells that is impermeable to both water and CO2. To enable CO2 entry into the leaf for photosynthesis, the epidermis is perforated by pores called stomata (Figure 1.5). As CO2 molecules diffuse inwards they encounter an opposite flux of H2O molecules rushing outwards that is three to four orders of magnitude stronger. This problem of transpirational water loss is a particular problem for plants in hot, dry climates, such as in most of Australia. Leaves control this gas exchange by adjusting the aperture of stomata which can vary within minutes in response to changes in several environmental variables including light, humidity and CO2 concentration (see Chapter 15 for more details). Air-spaces inside leaves are effectively saturated with water vapour (equivalent to 100% relative humidity at that leaf temperature) and because air surrounding illuminated leaves is almost universally drier, water molecules diffuse outwards down this concentration gradient from leaf to air.
Figp1.05.png
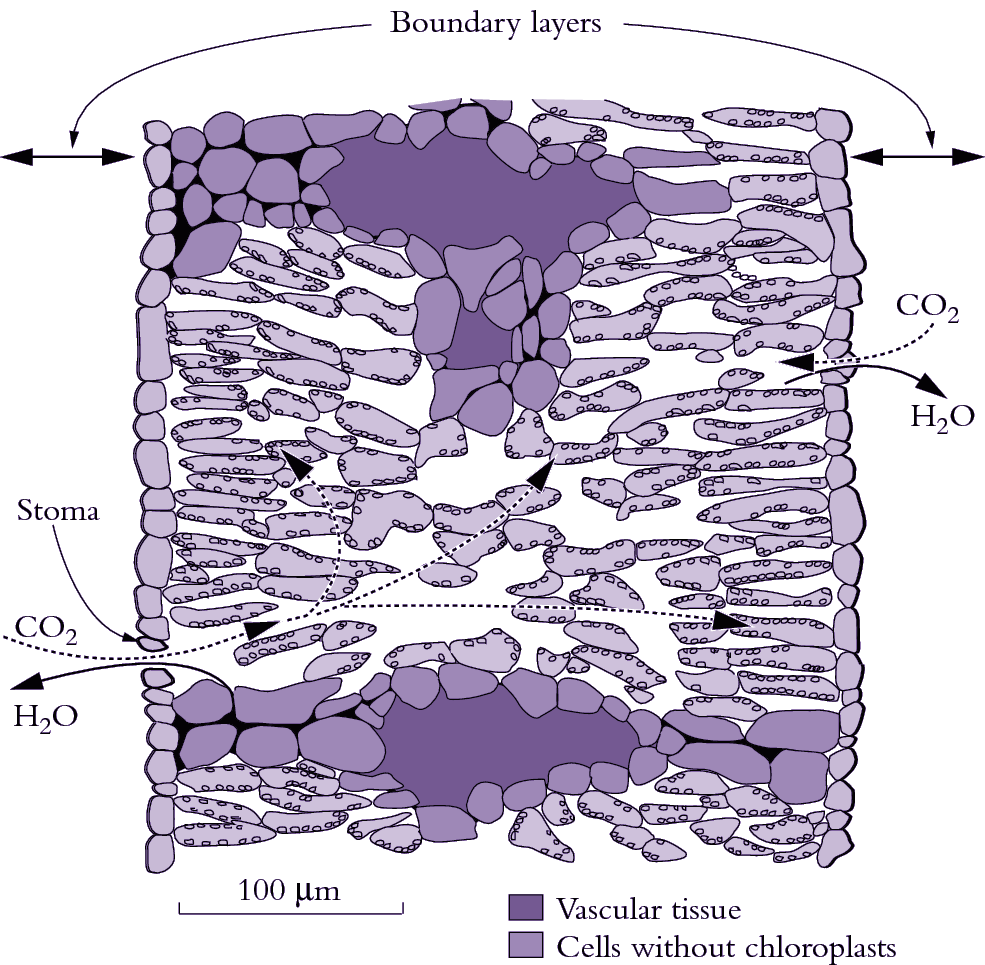
Figure 1.5 Diagram of a transverse section through an isolateral Eucalyptus pauciflora leaf which is normally pendulant. Palisade tissue occurs beneath both surfaces with spongy tissue and oil glands (not shown) in the middle. Putative pathways for diffusion of H2O out of substomatal cavities are shown by the solid curved arrows. CO2 diffuses inwards and H2O diffuses outwards in response to concentration differences between the leaf and air. Such gas exchange is restricted by a boundary layer (the unstirred layer of air at the leaf surface) and by stomata. One stoma is shown on each surface. CO2 diffusion continues inside the leaf mesophyll through airspaces between cells (curved dashed arrows) to reach cell walls adjacent to each chloroplast where CO2 dissolves and then diffuses into the chloroplast to reach the carboxylating enzyme Rubisco. Bundle sheath extensions (bottom of diagram) reach both epidermis and create an internal barrier to lateral diffusion. (Based on J.R. Evans et al., Planta 189: 191-200, 1993)
The diffusion pathway for H2O out of a leaf is usually divided into two parts, namely the boundary layer of still air at the leaf surface and stomatal pores (Figure 1.5). Boundary layer thickness depends on windspeed, leaf dimensions and the presence of surface structures (e.g. hairs in Figure 1.1). Positioning of stomata also varies between species. Leaves of terrestrial plants always have stomata on their lower (abaxial) surface but many species have stomata on both surfaces, especially if they have high photosynthetic rates and are in sunny locations such as pendulant leaves of eucalypts. Adaptations for arid environments include having surface structures like hairs and waxes, which increase the thickness of the boundary layer, and leaf rolling and encryption of stomata by placing them in crevices in the leaf surface. While these features restrict water loss, they also impose an increased resistance (decreased conductance) to CO2 uptake.
The flux of water escaping from a leaf, called transpiration rate, can be understood from Fick’s law. It depends on the product between conductance and the gradient in water vapour from the inside of the leaf to the surrounding air. The vapour pressure gradient depends on both the humidity of the surrounding air and leaf temperature. Dry air (low humidity), or hotter leaf temperatures will result in greater transpiration rates for a given conductance. Maximum leaf conductance depends on the number and size of stomata per unit leaf area which is a leaf property that becomes fixed during development. However, the aperture of stomata can be varied, so stomatal conductance can vary over the timescale of minutes. Stomatal conductance responds to light, CO2 and humidity. The sensitivity of a leaf to these variables is not fixed but can change over time in response to, for example, drought. Transpiration rate can be measured by a variety of means. With the availability of portable instruments, it is now most commonly obtained by measuring the increase in water vapour content of air from a leaf enclosed in a chamber. Stomatal conductance can then be calculated from Fick’s law by dividing the transpiration rate by the vapour pressure gradient between the leaf and the air.
CO2 molecules diffusing inwards from ambient air to chloroplasts encounter restrictions additional to boundary layer and stomata (Figure 1.5). CO2 must also diffuse from substomatal cavities throughout the mesophyll, dissolve in wet cell walls, cross the plasma membrane to enter the cytosol, diffuse into chloroplasts across a double membrane (outer envelope in Figure 1.7) and finally reach fixation sites within the stroma of those chloroplasts. The combination of these restrictions from intercellular airspace to the sites of fixation within chloroplasts has been termed mesophyll conductance.
There is considerable variation in leaf anatomy and hence potential restriction to CO2 diffusion, but in general leaves with high rates of photosynthesis tend to have more permeable leaves (e.g. tobacco in Figure 1.2) and this complex anatomy ensures a greatly enlarged surface area for diffusion across interfaces. Indeed the total mesophyll cell wall area can be 20 times that of the projected leaf surface.
Chloroplasts tend to be appressed against cell walls adjacent to intercellular spaces (Figure 1.2 C, D) which improves access to CO2, and they contain carbonic anhydrase which speeds up diffusion of CO2 by catalysing interconversion of CO2 and bicarbonate within the stroma of chloroplasts. Although CO2 rather than HCO3– is the substrate species for Rubisco, the presence of carbonic anhydrase enables bicarbonate ions, which are more abundant under the alkaline conditions (pH 8.0) that prevail inside chloroplasts, to diffuse to Rubisco in concert with diffusion of CO2. By sustaining a very rapid equilibration between CO2 and HCO3– immediately adjacent to active sites on Rubisco, carbonic anhydrase enhances inward diffusion of inorganic carbon.