1.0-Ch-Fig-1.1-v2.jpeg
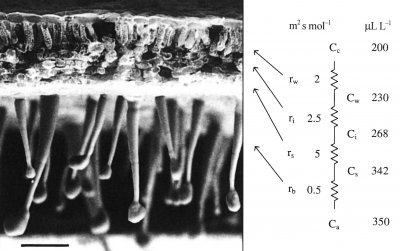
Figure 1.1 A scanning electron micrograph of an uncoated and rapidly frozen piece of tobacco leaf showing a hairy lower leaf surface and cross-sectional anatomy at low magnification. Notional values for resistances to CO2 diffusion are given in units of m2 s mol-1. Corresponding values for CO2 concentration are shown in µL L-1. Ci is routinely inferred from gas exchange measurements and used to construct A:Ci curves for leaf photosynthesis. Scale bar = 100 µm. (Image courtesy J-W. Yu and J. Evans)
In a typical herbaceous dicotyledon (Figure 1.1) lower leaf surfaces are covered with epidermal outgrowths, known to impede movement of small insects, but also contributing to formation of a boundary layer. This unstirred zone of air immediately adjacent to upper and lower epidermes varies in thickness according to surface relief, area and wind speed. Boundary layers are significant in leaf heat budgets and feature in the calculation of stomatal and mesophyll conductances from measurements of leaf gas exchange.
The diffusion of CO2 into leaves can be modelled using an analogue with electrical resistance (R) and conductance (the reverse of resistance), as in Figure 1.1, right hand side. This shows a series of resistances (r) that would be experienced by CO2 molecules diffusing from outside (ambient) air, through the boundary layer (b), the stomata (s), the intercellular airspaces (i), the cell walls and liquid phase (w) to fixed sites inside chloroplasts. These values emphasise the prominence of stomatal resistance within the series.
Corresponding values for CO2 concentration in ambient air (a), the leaf surface (s), the substomatal cavity (i), the mesophyll cell wall surface (w) to the sites of carboxylation with the chloroplasts (c) reflect photosynthetic assimilation within leaves generating a gradient for inward diffusion.
In transverse fracture as shown below in Figure 1.2(A) the bifacial nature of leaf mesophyll is apparent with columnar cells in the palisade layer beneath the upper surface and irregular shaped cells forming the spongy mesophyll below. Large intercellular airspaces, particularly in the spongy mesophyll, facilitate gaseous diffusion. The lower surface of this leaf is shown in Figure 1.2(B). On the left-hand side, the epidermis is present with its irregular array of stomata. Diagonally through the centre is a vein with broken-off hair cells and on the right the epidermis has been fractured off revealing spongy mesophyll cells beneath. Light micrographs of sections cut parallel to the leaf surface (paradermal) through palisade (C) and spongy (D) tissue reveal chloroplasts lying in a single layer and covering most of the internal cell wall surface adjacent to airspaces. Significantly, chloroplasts are rarely present on walls that adjoin another cell. Despite the appearance of close packing, mesophyll cell surfaces within the palisade layer are generally exposed to intercellular airspace. Inward diffusion of CO2 to chloroplasts is thereby facilitated.
Leaves that develop in sunny environments and have high photosynthetic capacities are generally thicker than leaves from shaded environments. This is achieved with more elongate cells within the palisade layer and/or several layers of cells forming the palisade tissue. Thicker leaves in a sunny environment enable more Rubisco to be deployed which confers a higher photosynthetic capacity. Fitting more Rubisco into a unit of leaf area with good access to intercellular airspace requires an increase in mesophyll cell surface which is possible by increasing the thickness of the mesophyll tissue and hence leaf thickness. A thicker leaf in sunny environments is energy effective because enough photons reach chloroplasts in lower cell layers to keep their Rubisco gainfully employed. By contrast, in a shaded habitat, less Rubisco is required for a leaf with lower photosynthetic capacity and this can be fitted into thinner leaves.
1.0-Ch-Fig-1.2.jpeg
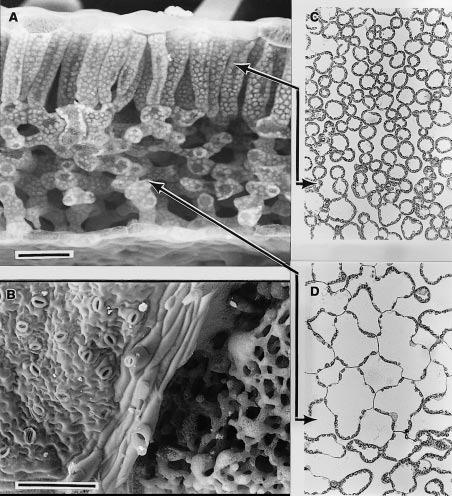
Figure 1.2 A scanning electron micrograph of an uncoated and rapidly frozen piece of tobacco leaf fractured in (A) to reveal columnar mesophyll cells of the palisade layer beneath the upper leaf surface and spongy mesophyll in the lower half. Chloroplasts can be clearly seen covering the inner faces of cell walls. Looking onto the lower surface (B), the epidermis and stomata are present on the left side of the vein, whereas the epidermis was fractured away on the right side, revealing spongy mesophyll tissue. Light micrographs (C, D) of sections cut parallel to the leaf surface are shown for palisade (C) and spongy mesophyll (D) with solid lines showing where the paradermal sections align with (A). Chloroplasts form a dense single layer covering the cell surfaces exposed to intercellular airspace, but are rarely present lining walls where two cells meet. Scale bar in (A) = 50 µm and (B) = 200 µm. (C) and (D) have same magnification as (A). (Images courtesy J. Evans and S. von Caemmerer)