For over a century, it has been known that short-term exposure to cold in measurements lasting minutes to a few hours results in reduced rates of respiratory CO2 release and O2 uptake. When measured over a range of moderate temperatures (e.g. 15-25oC), the relationship between respiration and temperature is near exponential, with respiration exhibiting short-term Q10 values near 2.0 (i.e. respiration increases two-fold for every 10oC increase in temperature). However, when viewed over a wider range of temperatures, the shape of the temperature response tends to be more dynamic, reflecting the fact that the functional form of the short-term temperature response curve of respiration departs significantly from a simple exponential. As a result, short-term Q10 values typically increase with short-term decreases in measuring, commonly reaching values >3.0 at temperatures in the 0-10oC range. Theory and empirical evidence suggests that the increasing temperature-sensitivity of respiration as measurement temperatures decrease is linked to shifts in the control exerted by substrate limitations at moderate-high temperature to maximum enzyme activity at low temperature. This is either because of the inhibitor effect of cold on potential enzyme activity per se (both in soluble and membrane-bound compartments) and/or limitations on the function of enzymes embedded in membranes at temperatures below the Tm. At moderately high temperatures (e.g. 25oC), respiratory flux is less limited by enzymatic capacity because of increases in the Vmax [i.e. maximal flux through the respiratory system as a whole, or parts thereof, in the absence of other limiting factors (e.g. substrate supply and adenylates)] of enzymes in soluble and membrane-bound compartments; here, respiration is likely to be limited by substrate availability and/or adenylates (in particular the ratio of ATP to ADP and the concentration of ADP per se, which are in turn influenced by the demand for respiratory energy by growth and cellular maintenance processes). Increased leakiness of membranes at temperatures above the Tm (particularly at high temperatures) could further contribute to substrate limitations. Changes in growth temperature that last several days can also alter the short-term Q10, with Q10 values also varying seasonally in some ecosystems.
A further factor that could contribute to variability in the sensitivity of respiration to low temperatures is the extent to which cold differentially suppresses activity by the two terminal pathways via which electrons are transferred to oxygen in the inner mitochondrial membrane [i.e. the phosphorylating cytochrome oxidase pathway (COP) versus the non-phosphorylating alternative oxidase pathway (AOP)]. To date, results from studies with intact tissues and isolated mitochondria from a range of plant species and organs have yielded conflicting conclusions, with some studies suggesting that the AOP might be less sensitive to cold that the COP, while others have reported the opposite or little difference between the temperature sensitivity of the two pathways. Thus, at this stage it is unclear to what extent differential temperature sensitivities of the AOP and COP play a role in influencing variations in the short-term Q10 of plant respiration.
Fig14.24.png
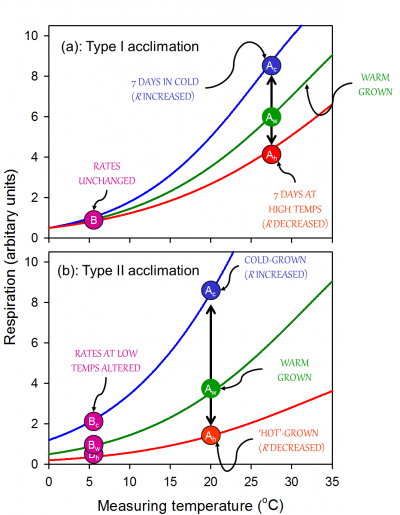
Figure 14.24. Theoretical examples of two types of respiratory thermal acclimation: (a) Type I and (b) Type II. (a) in Type I acclimation, changes in growth temperature result in changes in the Q10 of respiration with no change in the value of respiration at low temperatures (‘B’) (i.e. the intercept remains unchanged). Rather, changes in respiration are only observed at moderate to high temperatures (‘Ac’>’Aw’>’Ah’). Shifting to low growth temperatures for an extended period typically results in an increase in the Q10, whereas the Q10 decreases following shift to high growth temperatures. (b) Type II acclimation results in changes in respiration at both low and high temperatures (i.e. the overall elevation of the temperature response curve is affected). No changes in the Q10 of respiration are necessary for Type II acclimation. Type II acclimation will result in a greater degree of homeostasis of respiration than Type I acclimation. Source: Atkin and Tjoelker (2003)
With sustained exposure to cold, respiratory rates (when measured at a low temperature) start to recover quickly (within hours), and continue to increase as the plant acclimates. Often, the capacity for respiratory enhancement is relatively low in pre-existing tissues; here, acclimation is associated with a change in the rate of respiration primarily at moderate to high measuring temperatures, with little or no change in respiration at low measuring temperatures (i.e. Type I acclimation; Figure 14.24, reflecting a change in the availability of respiratory substrate and/or degree of adenylate restriction of respiration. Changes in gene expression may also occur, but are not essential for the overall change in respiratory flux. In other cases, acclimation is associated with an increase in the rate of respiration over a wide range of measurement temperatures (‘Type II acclimation’; Figure 14.24). Type II acclimation is likely associated with temperature-mediated changes in respiratory capacity that can be maximally realized through growth of new tissues with altered morphology and biochemistry. Increases in respiratory capacity in the cold appears to be altered as a result of increases in the density of mitochondria mitochondrial number per unit volume of tissue and/or amount of total protein invested in the respiratory chain. The observation that mitochondrial density is higher in in situ alpine than lowland plants further supports the notion that an increase in mitochondrial number is a driving force behind increased respiratory rate in cold-acclimated leaves. Intermediate cases of acclimation (i.e. between Types I and II) are likely, particularly in individual plants that experience long-term changes in temperature depending on the extent to which respiratory capacity is altered in pre-existing and newly formed leaves and roots. Another characteristic of acclimation (particularly Type II acclimation) is that it can result in respiratory homeostasis [i.e. identical rates of R in plants grown and measured in contrasting temperatures (Figure 14.24).
Previous work examining the impact of sustained exposure to cold on respiration has suggested that the AOP might play a critical role in the cold acclimation response. In some studies, alternative oxidase transcript abundance, protein abundance, and capacity have all been shown to increase following growth in the cold. In addition, there are examples where in vivo partitioning of electrons to the AOP has been shown to increase following sustained exposure to cold. Such studies have led to the proposal that cold induced increases in AOP activity function to prevent the over-reduction of the mitochondrial electron transport chain, and thus the accumulation of ROS, at low temperatures. However, in reality the response of the AOP to cold may be more complex. For example, in some cases, the recovery of AOP activity in cold acclimated plants is transient (e.g. reaching a maximum after few days of sustained cold treatment), while others have reported that re-establishment of respiratory flux in the cold is associated not with an increase in AOP capacity, but rather with an increase in energy-conserving COP capacity. Such variability in the AOP response suggests that different plant species employ different strategies for coping with cold.
Finally, consideration should be given to the fact that plant mitochondria possess two additional non-phosphorylating bypasses of the mitochondrial electron transport chain: the alternative NAD(P)H dehydrogenases (NDHs) and the uncoupling proteins (UCPs). Both of these proteins function to reduce the extent to which mitochondrial electron transport is coupled to the production of ATP. The NDHs oxidize matrix and cytosolic NAD(P)H, but do not contribute to proton pumping, while the UCPs facilitate proton flux back through the inner mitochondrial membrane, thereby partially dissipating the proton gradient across this membrane. Both the NDHs and the UCPs are thought to play a role in preventing oxidative stress, with sustained cold treatment increasing transcripts for both types of proteins. Moreover, there is growing evidence that transcript levels of these alternative respiratory bypasses exhibit coordinated increases in abundance following sustained cold treatments. Collectively, the above suite of modifications in metabolic processes contribute to the ability of many plants to cope with sustained exposure to low temperatures.