Fig6.17.png
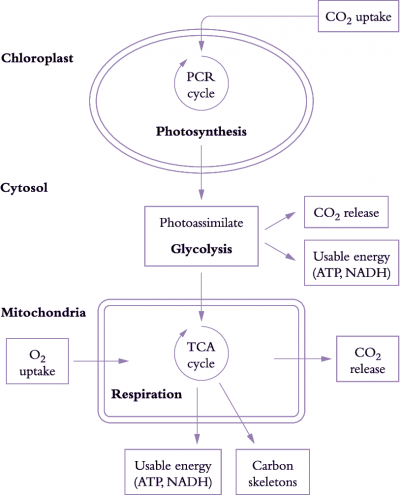
Figure 6.17. Simplified view of processes involved in carbon gain and generation of respiratory energy. The figure represents a mesophyll cell in a leaf. CO2 assimilated by chloroplasts produces carbon-rich compounds (photoassimilates) that are exported to the cytosol and mitochondria. CO2 is then produced during breakdown of these carbon-rich compounds by glycolysis and by mitochondrial respiration. Release of CO2 and uptake of O2 by mitochondria are coupled to production of usable energy (ATP, NADH). Carbon skeletons (necessary for protein synthesis) are also produced during mitochondrial respiration (Original drawing courtesy Owen Atkin)
(a)Photosynthesis and energy production
Photoassimilate is used to generate respiratory products needed for plant growth (Figure 6.17). Carbohydrate compounds produced from photosynthesis are exported from chloroplasts to the cytosol and mitochondria, and used to generate ATP, redox equivalents (in particular NADH) and carbon skeletons via glycolysis, mitochondrial tricarboxylic acid (TCA) activity and mitochondrial electron transport. Generation of these respiratory products results in CO2 loss during glycolysis and passage of metabolites around the TCA cycle.
(b)Respiration and energy utilisation
Energy (ATP and NADH) and carbon skeletons produced by mitochondrial respiration are used for various processes essential to growth, maintenance, nutrient uptake and transport within the plant.
Maintenance respiration represents the portion of respiratory CO2 release that is coupled to production of energy (ATP and reducing power) necessary for maintenance of chemical and electrochemical gradients across membranes, turnover of cellular constituents such as proteins, and processes involved in physiological acclimation to changing or harsh environments (Penning de Vries 1975). Energy needed for maintenance is determined by the specific costs of processes taking place and is generally regarded as proportional to tissue mass.
Protein turnover is an energy-intensive process accounting for 60–80% of maintenance respiration (Penning de Vries 1975). Demand for respiratory energy associated with protein turnover will depend on turnover rate, respiratory costs associated with turnover, as well as the total amount of proteins undergoing turnover. Enzymes such as nitrate reductase (a key enzyme involved in nitrogen assimilation) have a very high turnover rate (Amthor 1984). As a result, plants assimilating nitrate have higher maintenance requirements than ammonium-grown plants (Hansen 1979).
Translocation of photoassimilate is also a potentially expensive process that accounts for approximately 30% of total dark respiration in several starch-storing plant species and would represent a substantial drain on photo-assimilate that could otherwise go into storage organs (Table 6.6). Phloem loading and unloading is largely responsible for this high cost because transport of sugars between symplasm and apoplasm depends on cotransport of H+. Movement of H+ is in turn dependent on ATP being consumed in the symplasm (Chapter 5). Traffic in photoassimilate thus increases demand for maintenance respiration
Energy costs associated with nutrient aquisition are often very high because ions have to be transported across root cell membranes using active transport systems that require substantial amounts of ATP. Energy requirement for ion uptake will depend on several factors, including the degree to which absorbed nutrients are released back to the soil and the degree to which protons and anions are cotransported into roots.
Growth respiration covers synthesis of new biomass from photosynthate and mineral nutrients and is regarded as proportional to the rate at which new material is being formed. Specific respiratory costs associated with growth (i.e. construction cost) will depend to a large extent on the chemical composition of plant material and by implication the amount of energy embedded in these molecules (Table 6.7). Compounds with a high carbon concentration require more ATP and reducing power for their synthesis (Lambers and Poorter 1992). For example, biomass stored as lipid represents an investment of almost three times as much energy as would be required for storage of the same mass of non-structural carbohydrate. Plant growth analysis based on dry mass accumulation takes no account of such differences in chemical composition of end-products, so that comparisons of growth efficiencies based solely on RGR of biomass must be viewed circumspectly.
Construction cost, and thus growth respiration, also varies according to the chemical form of available nitrogen (e.g. N2, NO3– and/or NH4+) and sites of assimilation. Nitrogen reduction is an energetically expensive process, requiring considerable input of respiratory energy (e.g. ATP + reductant) and TCA cycle intermediates. Plants fixing atmospheric N2 in their roots demand much ATP, namely 12.5–26.5 mol ATP per mol of NH4+ produced, and a further 2.5–3.0 mol ATP for subsequent assimilation into nitrogen-based metabolites such as amino acids and proteins. NO3– reduction to NH4+ is cheaper, costing around 12 mol ATP per mol NH4+ produced.
Respiratory costs associated with NO3– assimilation can be substantially reduced if reduction of NO3– to NH4+ and subsequent assimilation of NH4+ into amino acids takes place in leaves. Reduction and assimilation of NO3– can then used excess photosynthetic reductant and ATP. Growth respiration associated with synthesis of nitrogen-based resources is thus greatly reduced by shoot assimilation of NO3–.