The glycolytic pathway involves the oxidation of the hexoses and hexose phosphates molecules produced from the breakdown of starch or sucrose to generate ATP, reductants and pyruvate (Figure 2.21).
Fig.2.21.png
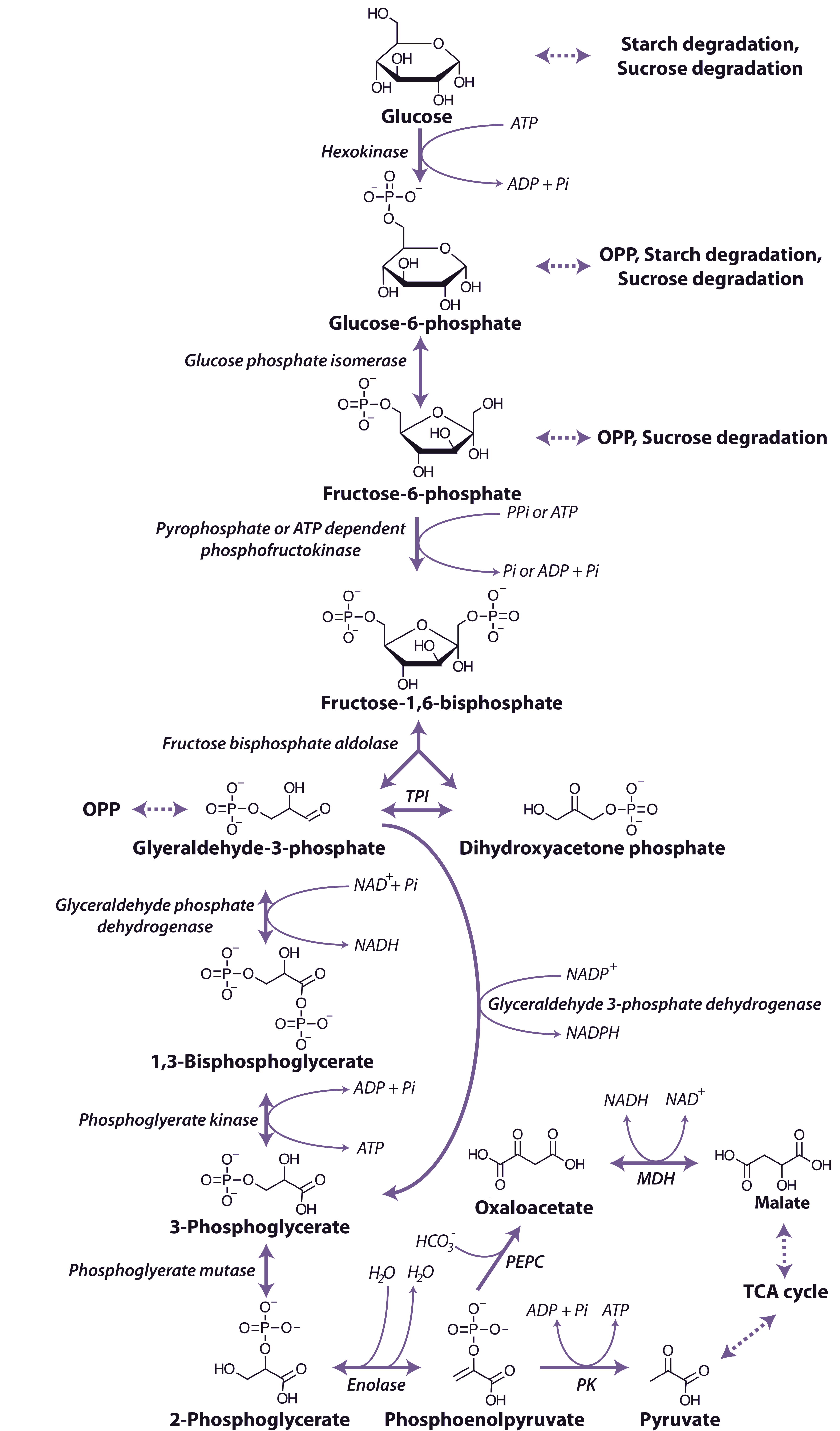
Figure 2.21 The glycolytic pathway. OPP, Oxidative pentose phosphate pathway; PPi, pyrophosphate, Pi, inorganic phosphate; TPI, triose phosphate isomerase; NAD+, nicotinamide adenine dinucleotide (oxidised); NADH, nicotinamide adenine dinucleotide (reduced); NADP+, nicotinamide adenine dinucleotide phosphate (oxidised); NADPH; nicotinamide adenine dinucleotide phosphate (reduced); ATP, adenosine triphosphate; ADP, adenosine diphosphate; MDH, malate dehydrogenase; PEPC, phosphoenolpyruvate carboxylase; PK, pyruvate kinase. (Original drawing courtesy Nicolas Taylor & Harvey Millar)
Regulation of PFK and PPi-PFK is achieved by a combination of mechanisms, including pH, the concentration of substrates and effector metabolites and changes in subunit association. Phosphoenolpyruvate (PEP) is a potent inhibitor of both of PFK and PPi-PFK, inhibiting at µM concentrations and Pi can activate the cytoplasmic PFK, whereas the plastidic form is slightly inhibited by Pi. A number of other effectors of PFK have been identified including ADP, 3-phosphoglycerate and phosphoglycolate as well as it ability to accept ribonucleoside triphosphates other than ATP as the phosphate donor. PPi-PFK, has a catalytic potential higher than that of PFK and is strongly activated by Fructose-2,6-bisphosphate, but has no effect on PFK.
Fructose-1,6-bisphosphate is cleaved by fructose bisphosphate aldolase to form glyceraldehyde-3-phosphate and dihydroxyacetone phosphate, and these triose phosphates can be interconverted in a reaction catalysed by triose phosphate isomerase. Glyceraldehyde-3-phosphate is oxidised to 1,3-bisphosphoglycerate by a nicotinamide adenine dinucleotide (NAD+)-dependent glyceraldehyde 3-P dehydrogenase. Glyceraldehyde 3-P dehydrogenase is sensitive to inhibition by the reduced pyridine nucleotide cofactor (NADH), which must be reoxidised to maintain the flux through the glycolytic pathway. A phosphate group is then transferred from 1,3-bisphosphoglycerate to ADP forming ATP and 3-phosphogylcerate by phosphoglycerate kinase. In the cytosol a bypass is present that can convert glyceraldehyde-3-phosphate directly to 3-phosphoglycerate without phosphorylation by a non-phosphorylating NADP dependent glyceraldehyde 3-phosphate dehydrogenase. The resulting 3-phosphoglycerate is then converted to phosphenolpyruvate (PEP) by the action of phosphoglycerate mutase and then enolase.
The end-products of glycolytic reactions in the cytosol are determined by the relative activities of the two enzymes that can utilise PEP as a substrate: pyruvate kinase, which forms pyruvate and a molecule of ATP, and PEP carboxylase, which forms oxaloacetate (Figure 2.21). Both of these reactions are essentially irreversible and there are fine controls that regulate the partitioning of PEP between these reactions. Pyruvate kinase is controlled post translationally by a partial C-terminal truncation which may yield altered regulatory properties and a phosphorylation and ubiquitin conjugation that targets the protein to the 26S proteasome for complete degradation, it is also inhibited by ATP. Whereas PEP carboxylase is inhibited by malate and thus its regulation is independent of cell energy status. The sensitivity of PEP carboxylase to malate is regulated by phosphorylation of a N-terminal serine of the enzyme, with the phosphorylated form less sensitive to malate inhibition. Oxaloacetate is then reduced by malate dehydrogenase to malate which, along with pyruvate, can be taken up into mitochondria and metabolised further in the TCA cycle (see below). The reduction of oxaloacetate in the cytosol could provide a cytosolic mechanism for oxidising NADH formed by glyceraldehyde 3-P dehydrogenase (Figure 2.21).
Another level of regulation of components of glycolysis is their physical location within the plant cell. Under conditions of high respiratory activity, a greater proportion of the cytosolic enzymes of glycolysis are present on the surface of mitochondria. In contrast when respiration is experimentally inhibited, a decrease in the association of glycolytic enzymes with the mitochondria is observed. It is likely that the glycolytic enzymes associate dynamically with mitochondria to support respiration and that this association restricts the use of glycolytic intermediates by competing metabolic pathways.