(a) Protecting nitrogenase from O2
A basic conflict arises in biological N2 fixation: nitrogenase is destroyed by O2, yet aerobic respiration is essential to sustain the high energy demand of N2 fixation. Nitrogen-fixing bacteria must be protected from O2, while a level of aerobic respiration occurs in the host cell cytoplasm. In cycads, cyanobacteria provide their own O2 protection. Nitrogenase is located in specialised cells (heterocysts) which have an O2-impermeable lining of glycolipid. An analogous structure (a vesicle) affords protection to nitrogenase in the microsymbiont Frankia within most actinorhizal nodules. In Parasponia and most caesalpinoid nodules the persistent infection threads provide O2 protection to nitrogenase (Sprent and Raven 1985).
There is one major problem with structures of ‘fixed’ resistance. As respiration rate varies (i.e. O2 flux), O2 concentration inside the structure must also vary: following Fick’s Law of diffusion, O2 flux into the nodule will change in proportion to the O2 concentration gradient at constant resistance. Free water bathing the nodule is equilibrated with the atmosphere (20.8% O2), therefore containing approximately 360 µM O2 in solution, while nitrogenase is destroyed by submicromolar concentrations of dissolved O2. For practical purposes then, an O2 gradient of 360 (outside) to 0 (inside) µM must be maintained. If respiration rate was halved without a change in resistance, the O2 concentration gradient would also halve from 360 to 180 µM. An O2 concentration of 180 µM O2 inside would destroy nitrogenase. So, resistance must vary too.
A legume-nodule cortex copes with variations in respiration rate by providing a variable level of O2 protection (Layzell and Hunt 1990). According to their model, a layer of cells adjacent to the infected zone either lacks radial intercellular spaces (preventing inflow of O2) or has intercellular spaces filled with water. The thickness of this layer could vary under osmotic control to set nodule permeability. Diffusivity of O2 through water is about 10,000 times slower than through air, so flooding of radial air spaces in the nodule cortex would be an effective way of decreasing O2 diffusion into infected tissue.
Any O2 leaking through this cortex can diffuse freely in the intercellular airspaces of infected tissue and dissolve in the cytoplasm of infected cells. O2 gradients which might be expected within infected cells because of rapid bacterial respiration are largely avoided by the presence of leghemo-globin (Lb) (a molecule similar to the hemoglobin in mammalian blood). O2 diffuses to Lb molecules where it is bound to form high concentrations of oxygenated Lb (estimated at 0.7 mM by Bergersen 1982). Effective nodules are pink because of oxygenated Lb (Figure 4.48); indeed this colour change can be used to estimate free O2 concentrations. Soybean nodules seem to regulate the free O2 in infected cells at between 5–60 nM (e.g. Layzell and Hunt 1990). Finally, residual O2 diffuses through the symbiosome to the bacteroids, supporting a level of aerobic respiration.
4.4-Ch-Fig-4.48.png
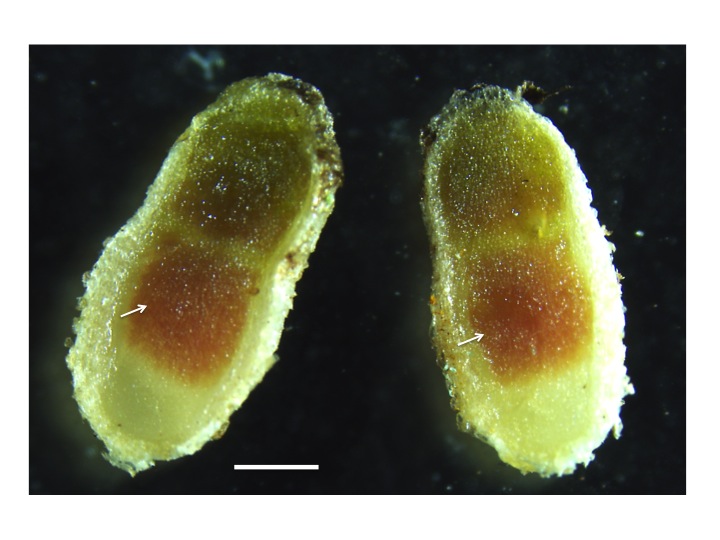
Figure 4.48 Leghemoglobin in nitrogen fixing nodules. The pink colour in the centre of the nodule (arrows) originates from the presence of leghemoglobin in the nitrogen-fixing zone of mature nodules. Leghemoglobin binds oxygen inside the nodules to protect the activity of nitrogenase. It also transports oxygen. Magnification bar = 1 mm. (Photograph courtesy U. Mathesius)
‘Conventional’ chemistry may not be appropriate when describing O2 movement in cells because O2 molecules in cellular compartments are so scarce. A sphere of 1 µm radius — roughly the size of a mitochondrion or bacterium — containing a solution with 10 nM O2 will contain only 24 molecules of O2.
(b) Carbon supply and nitrogen export
Nodules are metabolically highly active. A typical maximum rate of nitrogenase activity in soybean nodules, as measured by gas exchange (discussed below) is 300 µmol electron pairs g–1 (nodule) h–1. This value is useful to bear in mind when reading the literature about N2 fixation, with low values possibly indicating unhealthy or disturbed plants. As nitrogenase is at best 75% efficient, with respect to N2 fixation (Equation 2), this rate is equivalent to the fixation of some 150 µmol N g–1 (dry weight) h–1. Reduced nitrogen is exported from nodules to the host plant while carbon is imported into the nodule, supporting energy needs of fixation (through respiration) and providing carbon skeletons for packaging nitrogen as an organic molecule.
Photoassimilate (host to nodule) and nitrogen-based resources (nodule to host) must pass through the endodermis of nodule vascular bundles. Radial walls of this endodermis have Casparian bands and tangential walls have relatively few plasmodesmata, so this cell layer restricts apoplasmic and symplasmic flow of carbon into nodules and nitrogen out of nodules.
The transport of solutes, including C and N metabolites but also metal ions, e.g. Fe and Mo required for nitrogenase, are exchanged via both plant and bacterial transporters on the symbiosome and bacterial membrane, and this transport is carefully controlled to balance supply and demand (Figure 4.49; Udvardi and Poole 2013). Not all rhizobia that invade and inhabit legume nodules fix nitrogen efficiently, with the legume host able to restrict C flow to those nodules that do not fix sufficient N.
4.4-Ch-Fig-4.49.png
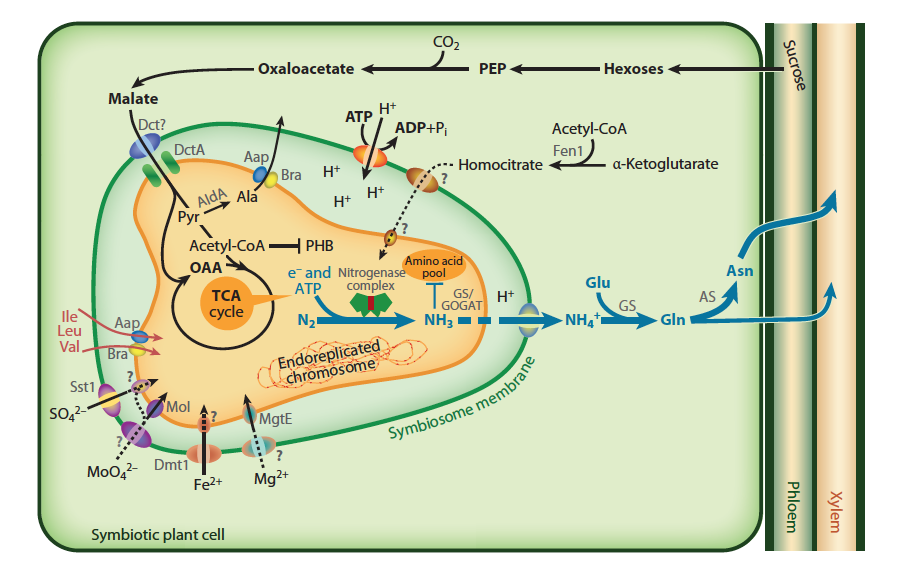
Figure 4.49 Transport and metabolism in an infected nodule cell. Sucrose from the shoot is converted to malate in the plant and imported across the symbiosome membrane and into bacteroids, where it fuels nitrogen fixation. The product of the nitrogen fixation is then exported back to the plant, where it is assimilated into asparagine (Asn) for export to the shoot (blue arrows). In many legumes, such as soybean, the export products are ureides instead of Asn. The plant must provide metals and ions to the bacteroid, although only some of the transport systems on the symbiosome and bacteroid membranes are defined. Many rhizobia lack the ability to make homocitrate or become symbiotic auxotrophs for supply of branched-chain amino acids and become dependent on the plant. (Reproduced with permission by Annual Reviews from M. Udvardi and P.S. Poole, Annu Rev Plant Biol 64: 781-805, 2013)
The concentration of nitrogenous solutes in the xylem apoplasm causes a hydrostatic pressure to develop, and this results in a mass flow of nodule xylem sap to adjacent roots. The water that accompanies sucrose entering the nodule as phloem sap is re-exported with assimilated nitrogen in the xylem. Nodules are thus analogous to ‘glands’ that secrete nitrogenous compounds.