Brian Atwell, Macquarie University, Australia
Flooding is an environmental hazard writ large across the agricultural regions of the planet. Major inundations constrain food production because few of the world’s crop species are hydrophytes (wetland plants). It is essential to discover mechanisms of tolerance that can be used as a basis for breeding and engineering flood tolerance in rice and also other crops.
Rice (Oryza spp.) is notable among the staple crops of the world for its extreme flood tolerance. Thus it has been a natural choice for discovery of flood-tolerance mechanisms. More specifically, the coleoptile of rice is subject in nature to extreme hypoxia or even anoxia and over thousands of years these organs have concentrated gene expression patterns that confer tolerance to O2 deficits.
The coleoptile, a small sheath that emerges from the seed (caryopsis) as a ‘proto-shoot’, is especially critical in this scenario because it grows preferentially while roots and leaves remain suppressed without O2 present. Coleoptiles also have special characteristics to make their growth energetically ‘cheap’. They consist of pre-formed cells and can increase in length by 30% each hour (Figure 1 of this Case Study) while the organ as a whole can elongate by 3 cm in a day under water. This illustrates that coleoptiles have evolved to be a perfect emergent organ, providing a conduit from anoxic soil/hypoxic floodwater to the water surface above. Reaching O2 is essential before energy deficits overwhelm the embryonic meristems. Indeed, this specific role of coleoptiles was called a ‘snorkel’ almost 50 years ago (Kordan, 1974) because it was realised that on reaching a source of O2, other organs like leaves and roots could grow.
CS_18.2-1.jpg
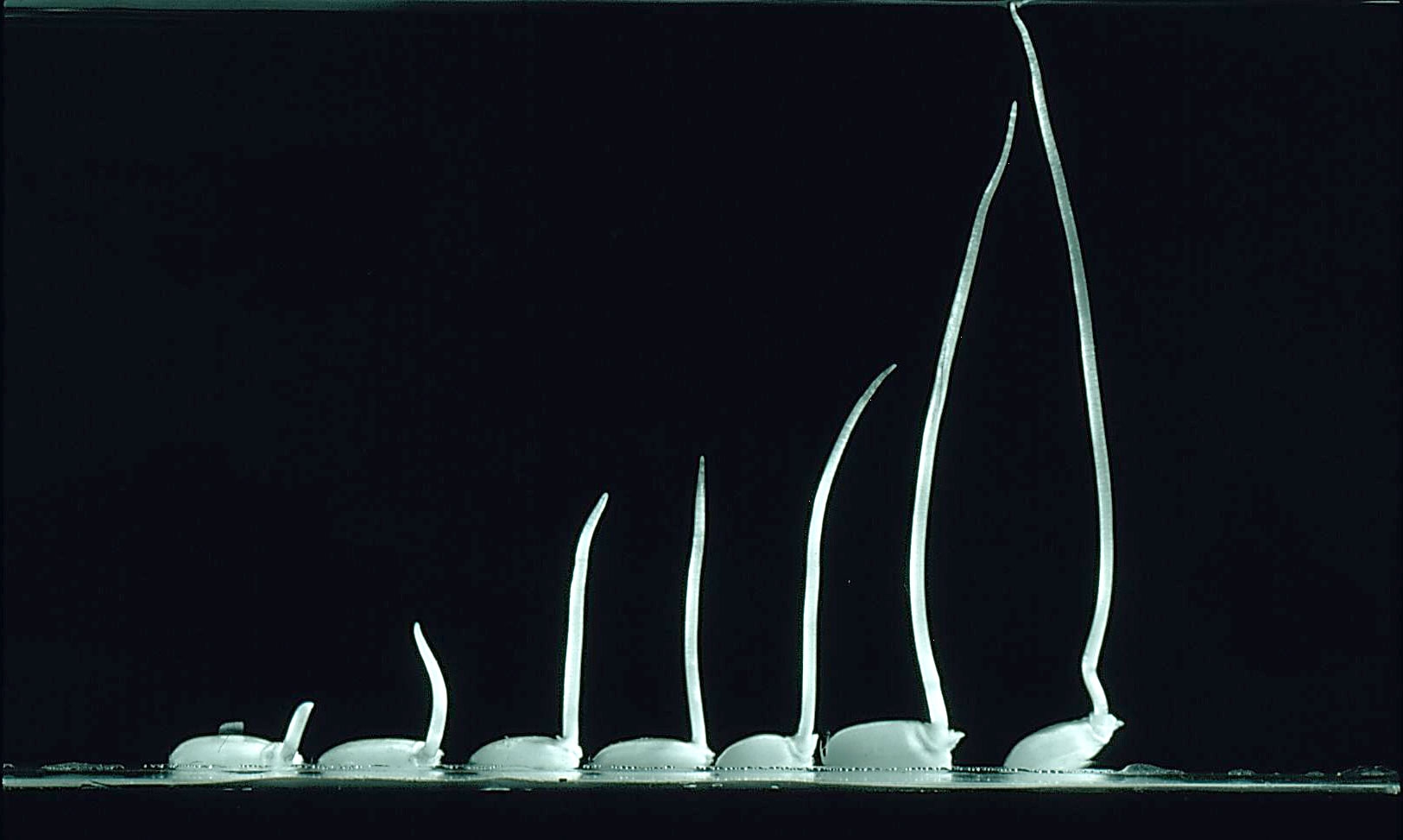
Figure 1. A time series of rice seedlings growing in hypoxic stagnant solution for 6 days. Note the lack of roots or true leaf. The seedling on the far right has commenced a new phase of development, with the production of a mesocotyl at the base of the coleoptile. This coleoptilar node is the first true shoot meristem. (Photograph courtesy of R. Oldfield and B.J. Atwell).
Fast coleoptile growth under water is stimulated by ethylene. The build up of this phytohormone causes coleoptiles of submergence-tolerant genotypes to ‘stretch’ towards to the water surface. Hence, rice coleoptiles constitute an example of the ‘escape’ strategy. As described in Case Study 1, a failure to perceive ethylene in shoots ensures survival in mature plants under long-term floods; the presence of the Sub1A gene induces dormancy and conserves carbohydrates. By contrast with that quiescent strategy, in areas such as river deltas with prolonged deep floods, internode elongation is beneficial for escape and with the discovery of two snorkel genes, has also been shown to be an ethylene-mediated phenomenon.
Mechanisms of anoxia tolerance in plants have been elucidated through studies of coleoptiles. Ethanolic fermentation can accelerate in anoxia because the Pasteur Effect speeds up glycolysis, using carbohydrates from the seed reserves. This provides a modestly better ATP supply than a non-hydrophyte could generate. Indeed, while ethanol was long been thought to be the dominant player in fermentation, other pathways involving haemoglobin and nitric oxide are now being invoked in energy production under anoxia. The realisation that maintenance and even growth in anoxia could be achieved by preserving critical energetically demanding reactions at the cost of non-essential reactions has opened new avenues of research. For example, we now know that protein synthesis becomes a very dominant use of ATP in anoxia while many other energised reactions succumb to energy shortages. Modification of the hierarchy of energy use occurs in animals and could be an evolutionary step unique to hydrophytic plants.
Ultimately, membrane potentials are vital for all living cells. The cost of maintaining potentials has been studied in depth, including under anoxia in coleoptiles. Proton pumping between compartments is vital and in anoxia, ATP-driven proton pumps become disabled. This induces a fall in the vital cytosolic pH but this fall is modest in rice whereas acidification can be lethal in intolerant species. Most remarkably, pyrophosphate is an alternative energy source to ATP for pumping protons in plants. This relatively ‘cheap’ energy source appears to energise proton pumping into the cell vacuole by a unique protein that is expressed most strongly in anoxia-tolerant cultivars of rice and is inducible within < 2 h of imposition of anoxia. Genetically knocking out the gene for this tonoplastic proton pump increases sensitivity to anoxia. Thus, genes encoding a pyrophosphate-driven pump at the tonoplast have been identified as targets for improving survival during flooding and have also recently been implicated in regulation of Na+ compartmentation crucial also to salinity tolerance.
This short account of some of the applications of rice coleoptiles to improve our understanding of stress tolerance illustrates the power of an ideal model. That one simple, undifferentiated organ could instruct us about hormone physiology, energy metabolism and membrane integrity is truly remarkable. This does not preclude the need for studies in more organisms, including wild plants or animals, but does reinforce the importance of model systems
Further reading:
Kordan HA (1974) The rice shoot in relation to oxygen supply and root growth in seedlings germinating under water. New Phytol 73: 695–697
Edwards JM, Roberts TH, Atwell BJ (2012) Quantifying ATP turnover in anoxic coleoptiles of rice (Oryza sativa) demonstrates preferential allocation of energy to protein synthesis. J Exp Bot 63: 4389-4402