Joseph Holtum1, Klaus Winter2 and Barry Osmond3
1Centre for Tropical Biodiversity and Climate Change, James Cook University, Australia; 2Smithsonian Tropical Research Institute, Balboa, Ancón, Republic of Panama; 3School of Biological Sciences, University of Wollongong, and Research School of Biology, Australian National University, Australia
Crassulacean acid metabolism (CAM) is a water-conserving mode of photosynthesis that, like C4 photosynthesis, is a modification of the C3 photosynthetic pathway fitted with a CO2 concentrating mechanism (CCM) that can increase the [CO2] around ribulose bisphosphate carboxylase/oxygenase (Rubisco) by more than 10-fold and suppress photorespiration. The overall energy demand of the CAM pathway is only about 10% more than that of C3 photosynthesis, as costs of the CCM machinery are partially offset by reducing photorespiration.
In C4 plants, as explained earlier in Section 2.2.2, this CCM is most commonly achieved by an “in-line turbocharger” based on initial CO2 fixation by phosphoenolpyruvate carboxylase (PEPC) into C4 acids in the cytoplasm of outer mesophyll cells. These acids diffuse rapidly to adjacent relatively CO2-tight bundle-sheath cells (Figure 2.31 right) where CO2 is released again. High [CO2] builds up in this spatially separated compartment where it is refixed by Rubisco.
2.0-Ch-Fig-2.31.png
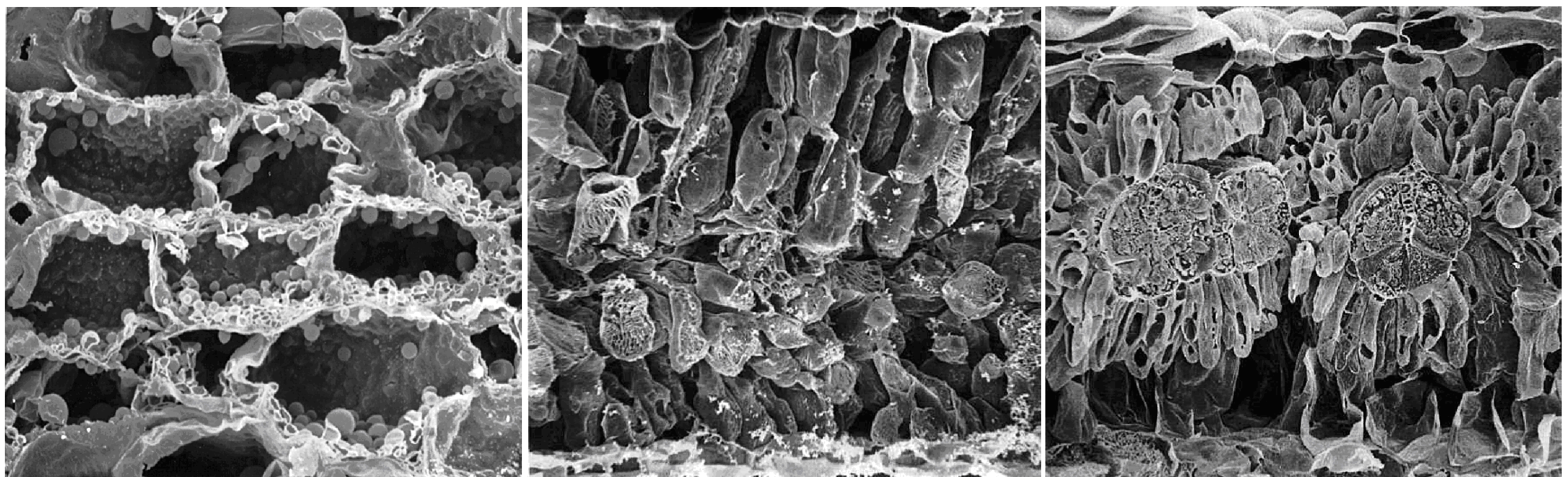
Figure 2.31 Leaf transverse sections of CAM versus C3 and C4 plants. Left: succulent CAM plant Kalanchoë daigremontiana. Centre: C3Atriplex hastata. Right: C4Atriplex spongiosa. Scanning electron micrographs at similar magnification. (K. daigremontiana image courtesy R.A. Balsamo and E.G. Uribe; Atriplex spp. images courtesy J. H. Troughton)
In CAM plants enzyme systems analogous to those in C4 plants achieve the same result through a “battery-like” dark accumulation of CO2 into the 2nd carboxyl group of malic acid (acidification phase) in the vacuole of large mesophyll cells (Figure 2.31 left). Malic acid can accumulate to very high concentrations, attaining concentrations of greater than 1 mole acid per litre in mesophyll cells of tropical tree-CAM plants (Clusia spp.). Indeed one can sometimes taste the acid, with acid taste-testing for the presence of CAM being possibly first recorded in Aloe sp. by Nehemiah Grew in 1682 and in field reports from India by Benjamin Heyne in 1815.
In the light, malic acid returns to the cytoplasm where it is rapidly decarboxylated (deacidification phase). The CO2 released, which accumulates to high internal [CO2] as stomata close, is refixed by Rubisco in chloroplasts of the same mesophyll cell where it is further assimilated by the photosynthetic carbon reduction (PCR) cycle (Figure 2.32).
Ultimately three of the four carbons recovered from the malic acid must be stored as starch and/or sugars in order to provide to PEPC the C3 substrate required for CO2 uptake during the following night. The fourth carbon, effectively that obtained from the atmosphere, is available for growth. Deacidification may generate high [CO2] behind closed stomata but photorespiration is not completely abolished (Lüttge 2002) since photosynthesis also generates high internal [O2]. While exploring Lake Valencia in Venezuela in 1800, Alexander von Humboldt measured elevated [O2] in bubbles streaming from the cut base of presumably CAM Clusia leaves standing in water in the light.
Of course by closing stomata in the light CAM plants minimize water loss when evaporative demand is highest (von Caemmerer and Griffiths 2009). The biomass production per unit water utilized in CAM was 6 times higher than for C3 plants and 2 times higher than for C4 plants when plants exhibiting all three photosynthetic pathways were grown together in a garden outdoors (Winter et al. 2005). The attribute of water-use efficiency undoubtedly contributes significantly to the success of CAM photosynthesis in nature, with CAM species outnumbering C4 species by about two to one. The paradoxes of CAM, a mode of photosynthesis that involves stomatal opening and CO2 uptake during the dark, continue to inform many aspects of plant biochemistry, physiology, ecology and evolution. This article draws heavily on two recent reviews (Borland et al. 2011; Winter et al. 2015).